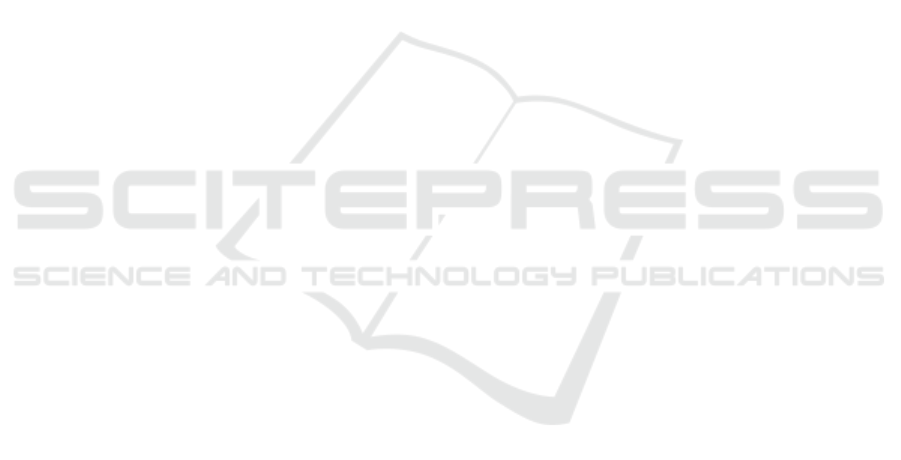
sensing features of WSS towards increased
immersive experiences. It is a mobile application
that uses a wind accessory, 3D audio and a Doppler
Effect simulation for the visualization and sensing of
georeferenced 360º hypervideos. The user evaluation
showed that the designed features increase the sense
of presence and immersion, and that users
appreciated them, finding all of them very useful,
satisfactory and easy to use. Users showed great
interest in the wind device, indicating this is a very
effective way to improve the realism of the
environment. Using 3D audio is a clear advantage
and it is an approach that does not require a new
infrastructure, as any pair of stereo headphones will
suffice and the sensors commonly available in
mobile devices allow detecting movement.
According to our tests, the sound sources distance to
the user’ head in the virtual sound space, which
should be a value comprised between 1 and 3
meters. The use of the Doppler Effect simulation,
when carefully manipulated as it was described, can
increase the users’ movement sensation, especially
in videos where there is a high degree of movement.
Next steps include: refining and extending our
current solutions, exploring further settings for
higher levels of immersion, like the CAVE and wide
screens, and other modalities to increase users’
engagement; exploring 3D audio capture to further
increase realism in the spatial audio feature;
considering georeferencing, ambient computing and
augmented reality scenarios, e.g. as access points to
videos shot in the same place at a different time, to
compare them ‘overlaid’, or access videos with
similar speed as the current speed experienced by
the user (e.g. while traveling on a train). This
concept can be extended for further filters (e.g.
access videos in same time of day, or with similar
weather conditions), relying on reality aid in finding
videos and feeling more immersed in the virtual
video being experienced.
ACKNOWLEDGEMENTS
This work is partially supported by FCT through
LASIGE Multiannual Funding and the ImTV
research project (UTA-Est/ MAI/0010/2009).
REFERENCES
Amselem D. (1995). A Window on Shared Virtual
Environments. Presence: Teleoperators and Virtual
Environments, 4(2):130–145.
Bleumers, L., Broeck, W., Lievens, B., Pierson, J. (2012)
Seeing the Bigger Picture: A User Perspective on 360°
TV. In Proc. of EuroiTV’12, 115-124
Cardin, S., Thalmann, D., and Vexo, F. (2007) Head
Mounted Wind. In Proc. of Computer Animation and
Social Agents. Hasselt, Belgium, Jun 11-13. 101-108.
Courtois, C., D’heer, E. (2012) Second screen appli-
cations and tablet users: constellation, awareness,
experience, and interest. EuroiTV’12, 153-156.
Dobler, D., and Stampfl, P. (2004) Enhancing Three-
dimensional Vision with Three-dimensional Sound. In
ACM Siggraph’04 Course Notes.
Douglas, Y., and Hargadon, A. (2000) The Pleasure
Principle: Immersion, Engagement, Flow. ACM
Hypertext’00, 153-160.
Ekman, P. (1992) Are there basic emotions?
Psychological Review, 99(3):550-553.
Lehmann, A., Geiger, C., Wöldecke, B. and Stöcklein, J.
(2009) Poster: Design and Evaluation of 3D Content
with Wind Output. In Proc. of 3DUI.
Mendes, M. (2010) RTiVISS | Real-Time Video Inter-
active Systems for Sustainability. Artech, 29-38.
Merer, A., Aramaki, M., Ystad, S., and Kronland-
Martinet, R. (2013) Perceptual Characterization of
Motion Evoked by Sounds for Synthesis Control Pur-
poses. In Trans. on Applied Perception, 10(1),1-23.
Moon, T., Kim, G. J., and Vexo, F. (2004) Design and
evaluation of a wind display for virtual reality. In
Proceedings of the ACM Symposium on Virtual
Reality Software and Technology.
Mourão, A., Borges, P., Correia, N., Magalhães, J. (2013)
Facial Expression Recognition by Sparse
Reconstruction with Robust Features. In Image
Analysis and Recognition. ICIAR'2013, LNCS, vol
7950, pp.107- 115.
Namezi, M., and Gromala, D. (2012) Sound Design: A
Procedural Communication Model for VE. In Proc. of
7th Audio Mostly Conference, Greece, 16-23.
Neng, L., and Chambel, T. (2010) Get Around 360º
Hypervideo. In Proc. of MindTrek, Tampere, Finland.
119-122.
Noronha, G., Álvares, C., and Chambel, T. (2012) Sight
Surfers: 360º Videos and Maps Navigation. In Proc.of
Geo MM'12 ACM Multimedia’12. Nara, Japan. 19-22.
Oliveira, E., Martins, P., and Chambel, T. (2011) iFelt:
Accessing Movies Through Our Emotions. In Proc. of
EuroITV'2011, Lisbon, Portugal, Jun 29-Jul 1. 105-
114.
Ramalho, J. and Chambel, T. (2013) Windy Sight Surfers:
sensing and awareness of 360º immersive videos on
the move. In Proc. of EuroITV 2013, Como, Italy.
107-116.
Ramalho, J. and Chambel, T. (2013) Immersive 360°
mobile video with an emotional perspective. In
Proc.
of the 2013 ACM international workshop on
Immersive media experiences at ACM Multimedia
2013, Barcelona, Spain. 35-40.
Slater, M., Lotto, B., Arnold, M.M., Sanchez-Vives, M.M.
(2009) How we experience immersive virtual
environments: the concept of presence and its
SensingImmersive360ºMobileInteractiveVideo
405