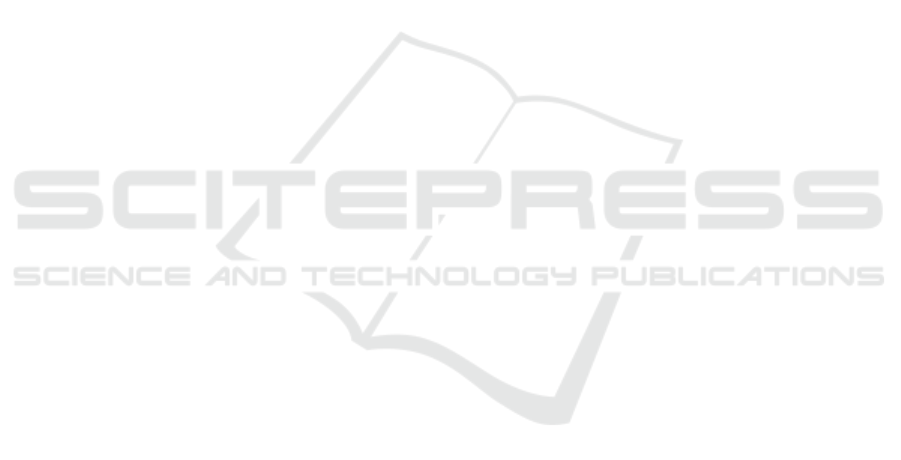
Study of the Evolution of Soot Formation using Laser-induced
Incandescence
Lincoln Tolomelli e Tolomelli
1
, Luiz Gilberto Barreta
1,2
,
Pedro Teixeira Lacava
1
and Dermeval Carinhana Jr.
1,2
1
Instituto Tecnológico de Aeronáutica, Pr. Mal Eduardo Gomes, 50 – Vila das Acácias, São José dos Campos, Brazil
2
Instituto de Estudos Avançados, Trevo Cel Av José A. A. do Amarante, n
o
1 – Putim, São José dos Campos, Brazil
Keywords: Biodiesel, LII, Diagnostic, Non-intrusive.
Abstract: Soot particles usually cause respiratory diseases and other problems to human health. To prevent or at least
reduces soot emissions it is necessary to know its formation mechanism. Laser-Induced Incandescence (LII)
has been used to detect soot and its precursors, known as polycyclic aromatic hydrocarbons (PAHs), in
diffusion flames. In this work, several mixtures of diesel/biodiesel blends were investigated using two laser
wavelengths, at 532 nm, which excites both soot and PAHs, and at 1064 nm, which excites only soot. Thus,
the difference of intensity between both LII signals provides the proportion of soot/PAHs in the irradiated
regions of flames, and it can be associated to the evolution of soot formation along the flame.
1 INTRODUCTION
Diesel engines are currently the largest source of
power generation in the planet, with employments in
transportation, mining machinery and other areas.
However, Diesel cycle is the major source of
atmospheric pollutants, as soot and PAHs
(polycyclic aromatic hydrocarbons) (Braun et al.
2003). Due to their small size, soot particles can
penetrate into the alveoli of the lungs and may cause
respiratory diseases (Lacava et al. 2010). Besides
health problems, the presence of soot represents an
important loss of energy in the thermodynamic cycle
and its generation is related with the incomplete
combustion of hydrocarbon fuels. Thus, the main
interest in the soot reduction concerns both to human
health and to environmental applications (Frenklach,
2002).
Soot are particles with size less than 0.1 µm, and
they are formed in the flame front, i.e., the region in
contact with the atmosphere air where burner
reactions of hydrocarbon fuels take place and solid
nuclei are generated. In this region, the complete
oxidation of hydrocarbon fuels is also observed,
mainly in poor fuel and high temperature flame
conditions (Williams, 1976).
Alternative fuels, such as biodiesel, have been
developed to reduce the emission of soot of the
transportation sector. Biodiesel is made by the
esterification of long-chain fatty acids derived from
vegetable oils or animal fat (Mello et al, 2001).
Biodiesel and its blends diesel/biodiesel present a
high efficient combustion and can replace mineral
diesel in compression ignition engines without
significant changing of performance (Altin et al,
2001).
The soot detection can be carried out using
several intrusive and non-intrusive techniques. One
of the most efficient one is the Laser-Induced
Incandescence (LII). LII is based on the temperature
rise of soot particles above the background
temperature by a high power incident laser. In this
condition, the irradiated particles emit radiation like
a blackbody, and the intensity of the continuous
radiation is proportional to soot fraction of volume
(Melton, 1984). When the laser pulse irradiates a
soot particle, four physical-chemistry phenomena
are observed in the particle (Figure 1): the
absorption of the radiation by the particle; the heat
conduction; the sublimation and, finally; the
blackbody emission or incandescence. Since this
method was first described, LII has been applied in
studies concerned to the spatial and the temporal
qualitative distribution of soot, the quantitative
determination of the soot fraction volume (Shadix
and Smith, 1996) and in the size evaluation of
primary particles (Mewes and Seitzman, 1997).
102
Tolomelli e Tolomelli L., Gilberto Barreta L., Teixeira Lacava P. and Carinhana Jr. D..
Study of the Evolution of Soot Formation using Laser-induced Incandescence.
DOI: 10.5220/0004714501020106
In Proceedings of 2nd International Conference on Photonics, Optics and Laser Technology (PHOTOPTICS-2014), pages 102-106
ISBN: 978-989-758-008-6
Copyright
c
2014 SCITEPRESS (Science and Technology Publications, Lda.)