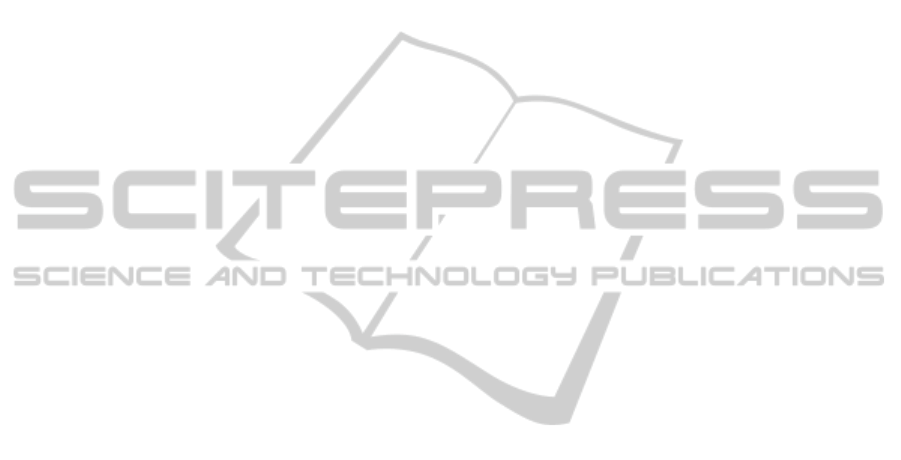
Temperature Sensing Fiber Bragg Gratings, IEEE
Photon. Technol. Lett., vol. 11, no. 9, pp. 1159-1161.
Gusarov, A. I., Berghmans, F., Fernandez, A. F., Deparis,
O., Defosse, Y., Starodubov, D., Decréton, M., Mégret,
P., and Blondel, M., 2000. Behavior of Fiber Bragg
Grating Under High Total Dose Gemma Radiation,
IEEE Trans. Nucl. Sci., vol. 47, no. 3, pp. 688-692.
Fernandez, A. F., Brichard, B., Berghmans, F., and
Decréton, M., 2002. Dose-Rate Dependencies in
Gamma-Irradiated Fiber Bragg Grating Filters, IEEE
Trans. Nucl. Sci., vol. 49, no. 6, pp. 2874-2878.
Gusarov, A., Kinet, D., Caucheteur, C., Wuilpart, M., and
Mégret, P., 2010. Gamma Radiation Induced Short-
Wavelength Shift of the Bragg Peak in Type I Fiber
Gratings, IEEE Trans. Nucl. Sci., vol. 57, no. 6, pp.
3775-3778.
Gusarov, A., Vasiliev, S., Medvedkov, O., Mckenzie, I.,
and Berghmans, F., 2008. Stabilization of fiber Bragg
Gratings against Gamma Radiation, IEEE Trans. Nucl.
Sci., vol. 55, no. 4, pp. 2205-2212.
Evans, B. D., 1998. The Role of Hydrogen as a Radiation
Protection Agent at Low Temperature in a Low-OH,
Pure Silica Optical Fiber, IEEE Trans. Nucl. Sci., vol.
35, no. 6, pp. 1215-1220.
Iino, A. and Tamura, J., 2010. Radiation Resistivity in
Silica Optical Fibers, J. Lightwave Technol., vol. 6,
no. 2, pp. 145–149.
Sanada, K., Shamoto, N., and Inada, K., 1994. Radiation
Resistance of Fluorine-doped Silica-core Fibers, J.
Non-Cryst. Solids, vol. 179, no. 4, pp. 339-344.
Henschel, H., Kuhnhenn, J., and Weinand, U., 2005.
Radiation Hard Optical Fibers, in Proceedings of
Optical Fiber Communication Conference, (Academic,
Anaheim, California, 2005), OThI1, pp. 1-3.
Nagasawa, K., Hoshi, Y., Ohki, Y., and Yahagi, K., 1985.
Improvement of Radiation Resistance of Pure Silica
Core Fibers by Hydrogen Treatment, Jpn. J. Appl.
Phys., vol. 24, no. 9, pp. 124-1228.
Kakuta, T., Shikama, T., Narui, M., and Sagawa, T., 1998.
Behavior of Optical Fibers under Heavy Irradiation,
Fusion Eng. Des., vol. 41, no. 1, pp. 201-205.
Dianov, E. M., Golant, K. M., Khrapko, R. R., and
Tomashuk, A. L., 1995. Nitrogen Doped Silica Core
Fibers: A New Type of Radiation-resistant Fiber,
Electron. Lett., vol. 31, no. 17, pp. 1490-1491.
Ju, S., Watekar, P. R., and Han, W.-T., 2010. Enhanced
Sensitivity of the FBG Temperature Sensor Based on
the PbO-GeO
2
-SiO
2
Glass Optical Fiber, J.
Lightwave Technol., vol. 28, no. 18, pp. 2697–2700.
Hill, K. O. and Meltz, G., 1997. Fiber Bragg Grating
Technology Fundamentals and Overview, J.
Lightwave Technol., vol. 15, no. 8, pp. 1263–1276.
Cavaleiro, P. M., Araújo, F. M., Ferreira, L. A., Santos, J.
L., and Farahi, F., 1999. Simultaneous Measurement
of Strain and Temperature Using Bragg Gratings
Written in Germanosilicate and Boron-Codoped
Germanosilicate Fibers, IEEE Photon. Technol. Lett.,
vol. 11, no. 12, pp. 1635-1637.
Gamma-rayDose-rateDependenceofFiberBraggGratingInscribedGermano-silicateGlassOpticalFiberwith
Boron-dopedInnerCladding
113