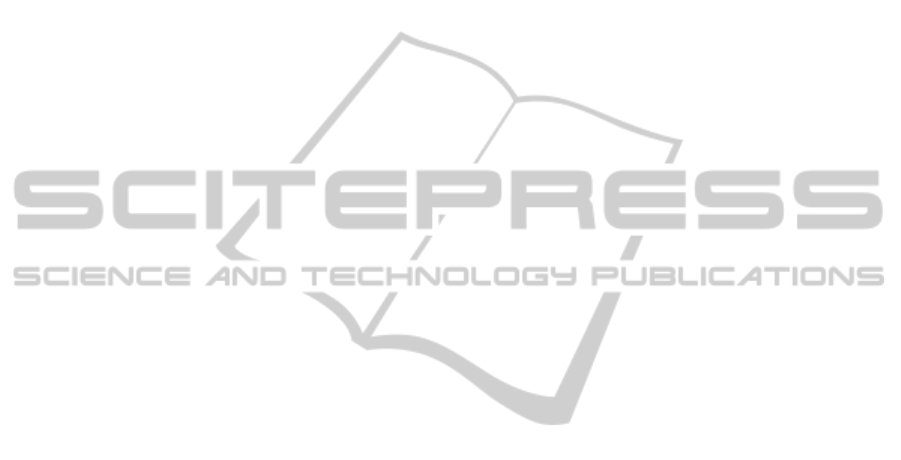
What is the interpretation of the psychosomatic
state of the subject when both heart rate and stress
level are increasing simultaneously?
Is there a correlation between events that occur
during the interaction with the system and the
aforementioned quantities?
Would the combinations provided by the two
states of each of the measured quantities show a
predictable pattern in response to certain emotional
events?
Could this system produce an adequately reliable
mechanism to map a psychosomatic condition onto
a descriptive model that would ideally be in
accordance with one of the established models of
affect, namely Russell’s (1980, 2003) circumplex
model?
Finally, can this system be used efficiently as a
component of an intelligent interface?
The structure of this paper is as follows: a brief
description regarding the biofeedback principles, our
purpose built electronic device and our system setup
is given. Subsequently, the experimental design
methodologies adopted and the development of the
front-end interface are outlined. Presumptions,
limitations, and predictions are clarified in detail in
the psychosomatic analysis section, followed by a
conclusive interpretation of our experimental data
and suggestions as to how the system may be
improved and used in broader areas of interactive
applications.
2 BIOFEEDBACK ACQUISITION
Derived from basic principles of biofeedback
measurements (Venables & Christie, 1980),
psychological stress is expressed in the human body
by a physiological response of brain induced
vasodilatation of sweat glands of the skin. This
alteration causes measurable changes in electrical
skin conductance that produces quantifiable
indicators of stress levels. Our purpose-built
electronic device used for the acquisition of HR and
stress measurements (SC) has been presented in
previous publications of the authors (Psaltis,
Mourlas, 2011). HR is acquired based on the
principle of Infrared Spectroscopy, effectively using
as sensing elements two deflecting infrared sensors
acquiring HR pulses, for optimised error
cancellation and a more reliable reading. For stress
measurement the skin conductance method has been
adopted as opposed to skin resistance, thus
providing a more reliable indication of the measured
quantity by eliminating inaccuracy problems due to
perspiration on the part of the subject.
Stress level is identified by two silver-silver
chloride contact rings measuring skin conductance,
placed on the sides of a computer mouse and at the
points where the thumb and middle fingers are
resting during the typical use of the mouse by a user.
The two HR sensors are situated in the centre of the
SC rings. Hardware redundancy is embedded in the
system allowing for two channel acquisition of
signals of HR and EC simultaneously, eliminating
artefacts and discontinuities caused by movement of
the fingers unless contact with the mouse is
completely lost. In case of lost contact the system
preserves the trait values until the next valid
measurement is detected. Minute losses of contact
with the sensors or erratic movement of the fingers
onto the mouse could produce an error in HR
reading in the scale of milliseconds between pulse
readings, while the SC measurement is unaffected.
Considering that our system does not take into
account detailed pulse shape and cardiac
arrhythmias, but is instead designed for detecting
heart rate and heart rate variability, this error is
within acceptable margins of tolerance of the
system, as it produces an estimated attenuation of
±0.18 of a pulse per reading. The mouse, including a
signal preconditioning circuit, is connected to a
computer via an electronic interfacing circuit that
filters and conditions the above primary signals and
converts them into a form that can be read by the
computer via the two channel audio input. A
purpose-built software suite comprising the
appropriate components required for a system
configuration console, signal processing and display
is the final component of the system. The use of this
console is essential for the initial settings required,
such as time interval between measurements, audio
card selection and settings, as well as data storage
configuration.
HR detection and correction, as well as SC auto-
calibration algorithms, have been implemented,
providing a relative baseline for each subject
independent of the actual stress levels of individuals.
Since our interest is not exactly how much stress is
the user experiencing but instead the state of stress
in relation to the previous level of stress, we devised
a method for auto calibration, envisaging our
reference point (“baseline”) at the mid distance of
the difference between highest and lowest measured
stress value weighed by the trait values. The baseline
is continuously updated based on the measurements
and the deviation from the mean value of SC (“tonic
level”). This was important primarily because it
PhyCS2014-InternationalConferenceonPhysiologicalComputingSystems
308