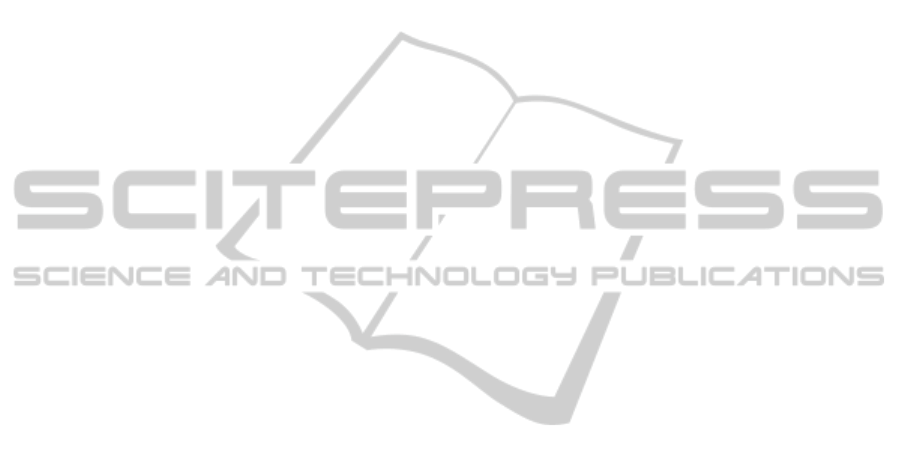
or algorithm design or by creating electrodes with
different contact properties. Furthermore, to give
a better characterization of the sensors, studies on
contact impedance variations over time should be
carried out. Electrodes that have shorter settling time
and that do not suffer from signal drifts are needed to
permit a faster, more reliable signal acquisition while
eliminating the undesired frequency components.
Due to the limitations of the proposed framework
and study design, conclusions regarding the relation
between signal quality and skin-electrode contact
properties are difficult to formulate. Future studies
should include a larger number of participants, both
male and female, for a more thorough, unbiased
analysis. During some of the experimental sessions,
gel electrodes were disconnected or made a poor
contact with the scalp of the participant. Also, due
to the constraints imposed by the rigid headset used
for dry electrode positioning, proper contact for dry
electrodes was not always obtained. Variability in the
size and head shape of the participants combined with
the different hair types, made the contact between
the skin and the electrode pins difficult. Results also
indicate that the reported outliers in signal quality
might be related to a bad contact of the electrodes
to the skin and not to the high skin-electrode contact
impedance magnitude.
Improvements to the proposed evaluation
framework should include a change in the stimulation
frequency of the SSVEP protocol. To avoid
interference from the strong low frequency
components, the alpha band frequency range
and the frequencies introduced by the walking
paradigms, a flickering frequency higher than 13Hz is
recommended. Also, placing the recording electrodes
above the occipital lobe would permit the capture of
a stronger SSVEP response as this site is closer to the
visual cortex. A protocol for eliciting event-related
potentials (ERPs) should also be included in the
evaluation as ERPs are often used in BCIs and
psychological studies. Moreover, the ERPs have a
very low amplitudes and thus are very sensitive to
different sources of interference.
Overall, better EEG systems and electrode
designs are needed to permit the usage of dry
electrode technology in a wide range of applications
requiring high quality signals. The skin-electrode
contact impedance broadly characterizes the interface
between the electrode and the skin. To allow the
design of better performing electrodes, more work
needs to be carried out in identifying the exact contact
characteristics that have the largest impact on signal
quality. Some of the approaches that could assist
in this process involve studying the skin-electrode
interface by analyzing the real and imaginary parts of
the impedance signal or by creating models of this
interface.
REFERENCES
Acunzo, D., Mackenzie, G., and Rossum, M. v. (2012).
Systematic biases in early ERP and ERF components
as a result of high-pass filtering. Journal of
Neuroscience Methods, 209:212–218.
Brainard, D. H. (1997). The Psychophysics Toolbox.
Spatial Vision, 10:433–436.
Chen, Y.-H., de Beeck, M. O., Vanderheyden,
Luc Mihajlovi
´
c, V., Grundlehner, B., and van
Hoof, C. (2013). Comb-Shaped Polymer-Based Dry
Electrodes for EEG/ECG Measurements with High
User Comfort. In 35th Annual International IEEE
EMBS Conference.
Chi, Y., Wang, Y.-T., Wang, Y., Maier, C., Jung, T.-P., and
Cauwenberghs, G. (2012). Dry and Noncontact EEG
Sensors for Mobile Brain-Computer Interfaces. IEEE
Transactions of Neural Systems and Rehabilitation
Engineering, 20(2):228–235.
Estepp, J., Monnin, J., Christensen, J., and Wilson,
G. (2005). Evaluation of a Dry Electrode
System for Electroencephalography: Applications
for Psychophysiological Cognitive Workload
Assessment. In Proceedings of the 11th International
Conference on Human Computer Interaction, Las
Vegas, Nevada, USA.
Estepp, J. R., Christensen, J. C., Monnin, J. W., Davis,
I. M., and Wilson, G. F. (2009). Validation of a
Dry Electrode System for EEG. In Proceedings
of the Human Factors and Ergonomics Society 53rd
Annual Meeting, pages 1171–1175, San Antonio,
Texas, USA.
Ferree, T. C., Luu, P., Russell, G. S., and Tucker, D. M.
(2001). Scalp electrode impedance, infection risk, and
EEG data quality. Clinical neurophysiology : official
journal of the International Federation of Clinical
Neurophysiology, 112(3):536–44.
Gargiulo, G., Calvo, R., Bifulco, P., Cesarelli, M., and
Ohamed, A. (2010). A New EEG recording system
for passive dry electrodes. Clinical Neurophysiology,
121:686–693.
Guevara, M. A. and Corsi-Cabrera, M. (1996). EEG
coherence or EEG correlation? International Journal
of Psychophysiology, 23:145–153.
Lin, C.-T., Wu, R.-C., Liang, S.-F., Chao, W.-H., Chen,
Y.-J., and Hung, T.-P. (2005). EEG-Based Drowsiness
Estimation for Safety Driving Using Idependent
Component Analysis. IEEE Transactions on Circuits
and Systems, 52(12):2726–2738.
Mihajlovi
´
c, V., Garcia-Molina, G., and J., P. (2012).
To What Extent Can Dry and Water-based EEG
Electrodes Replace Conductive Gel Ones? In
BioDevices conference, Vilamoura, Algarve, Portugal.
SignalQualityinDryElectrodeEEGandtheRelationtoSkin-electrodeContactImpedanceMagnitude
21