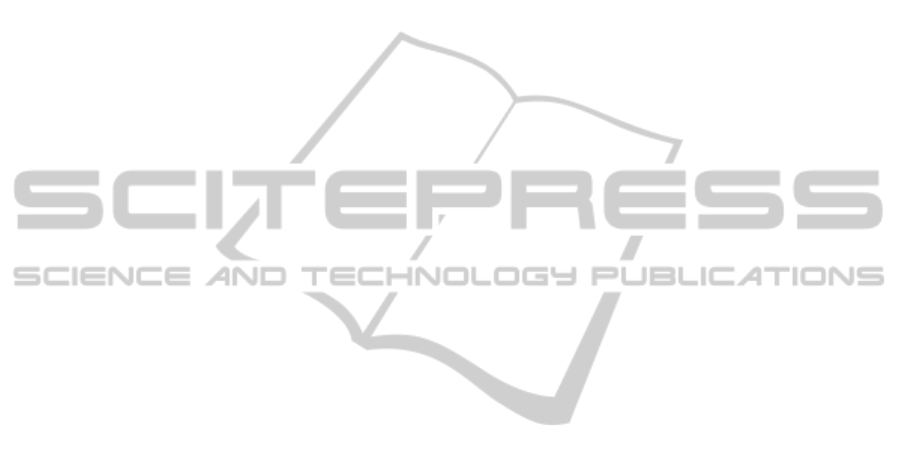
acquisitions present significant differences between
both groups of patients, as already proved by previ-
ous publications (Fisher et al., 2012). Results from
the group of patients present no significant differ-
ences from the results obtained for contra lateral ac-
quisitions while the group of subjects, presents higher
values of PLF and coherence for all frequencies, es-
pecially for beta-band. So, it is possible to assume
that while control subjects present no neuronal or
muscular anomalies and therefore higher synchrony
for beta-band, patients with ALS do not present syn-
chrony in any frequency, specially for beta-band. All
results allow to conclude that ipsilateral analysis is
a good measure of corticospinal pathways integrity.
Frequency spectrums were performed for contra lat-
eral acquisitions and it was demonstrated that beta-
band frequencies are present in instants of steady con-
traction. But, as proved by coherence and PLF analy-
sis beta-band frequencies in contralateral acquisitions
was not present in neither for patients or control sub-
jects. Neither differences or significance values were
found for both control and patients. Therefore, it is to
conclude that contra lateral frequency analysis is not a
good marker for beta-band frequencies studies. Com-
paring both methods used to study frequency domain,
PLF analysis may be seen as a more practical method
since it requires smaller sections of data, compared
to coherence. On the other hand, PLF can only be
analysed for a specific value of frequency, while co-
herence is analyzed for the range of frequencies pre-
sented on data. To conclude, as further work is sug-
gested to rely on the possibility of using PLF analysis
to observe beta-band and assess about neuronal path-
ways integrity in ipsilateral acquisitions. Therefore, it
seems possible that studying PLF serves as a method
of diagnosing ALS.
REFERENCES
Almeida, M., Vig
´
ario, R., and Bioucas-Dias, J. (2011).
Phase locked matrix factorization. In Proc. of the EU-
SIPCO Conference.
Baker, S. N., Chiu, M., and Fetz, E. E. (2006). Afferent
encoding of central oscillations in the monkey arm.
Journal of neurophysiology, 95(6):3904–3910.
Cordivari, C., Lees, A. J., Misra, V. P., and Brown, P.
(2002). Emg–emg coherence in writer’s cramp. Move-
ment disorders, 17(5):1011–1016.
de Carvalho, M. (2012). Testing upper motor neuron func-
tion in amyotrophic lateral sclerosis: the most difficult
task of neurophysiology. Brain, 135(9):2581–2582.
Farmer, S. F., Gibbs, J., Halliday, D. M., Harrison, L. M.,
James, L. M., Mayston, M. J., and Stephens, J. A.
(2007). Changes in emg coherence between long and
short thumb abductor muscles during human develop-
ment. The Journal of physiology, 579(2):389–402.
Fisher, K. M., Zaaimi, B., Williams, T. L., Baker, S. N.,
and Baker, M. R. (2012). Beta-band intermuscular
coherence: a novel biomarker of upper motor neu-
ron dysfunction in motor neuron disease. Brain,
135(9):2849–2864.
Grosse, P., Cassidy, M., Brown, P., et al. (2002). Eeg-emg,
meg-emg and emg-emg frequency analysis: physi-
ological principles and clinical applications. Clin-
ical neurophysiology: official journal of the In-
ternational Federation of Clinical Neurophysiology,
113(10):1523.
Grosse, P., Edwards, M., Tijssen, M., Schrag, A., Lees,
A. J., Bhatia, K., and Brown, P. (2004). Patterns of
emg–emg coherence in limb dystonia. Movement dis-
orders, 19(7):758–769.
Halliday, D. M., Conway, B. A., Farmer, S. F., and Rosen-
berg, J. R. (1998). Using electroencephalography
to study functional coupling between cortical activity
and electromyograms during voluntary contractions in
humans. Neuroscience letters, 241(1):5–8.
Jackson, A., Spinks, R., Freeman, T., Wolpert, D., and
Lemon, R. (2004). Rhythm generation in monkey mo-
tor cortex explored using pyramidal tract stimulation.
The Journal of Physiology, 541(3):685–699.
Kiernan, M. C., Vucic, S., Cheah, B. C., Turner, M. R.,
Eisen, A., Hardiman, O., Burrell, J. R., and Zoing,
M. C. (2011). Amyotrophic lateral sclerosis. The
Lancet, 377(9769):942–955.
Klimesch, W., Freunberger, R., Sauseng, P., and Gruber, W.
(2008). A short review of slow phase synchronization
and memory: evidence for control processes in differ-
ent memory systems? Brain research, 1235:31–44.
Marsden, J., Ashby, P., Limousin-Dowsey, P., Rothwell, J.,
and Brown, P. (2000). Coherence between cerebellar
thalamus, cortex and muscle in man cerebellar thala-
mus interactions. Brain, 123(7):1459–1470.
Nishimura, Y., Morichika, Y., and Isa, T. (2009). A sub-
cortical oscillatory network contributes to recovery
of hand dexterity after spinal cord injury. Brain,
132(3):709–721.
BIOSIGNALS2014-InternationalConferenceonBio-inspiredSystemsandSignalProcessing
170