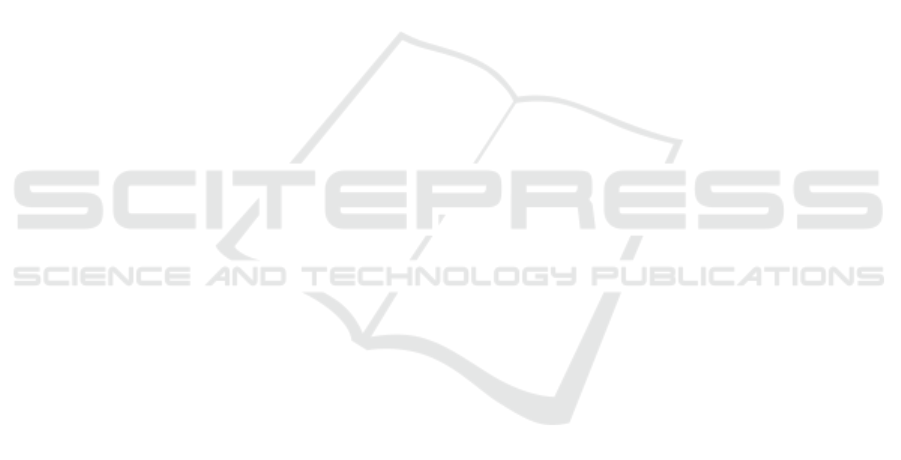
Molecular Beam Epitaxy of (Er
x
Sc
1-x
)
2
O
3
on Si(111)
for Active Integrated Optical Devices
H. Omi
1,2
, T. Tawara
1,2
, T. Hozumi
3
, R. Kaji
3
, S. Adachi
3
, H. Gotoh
1
and T. Sogawa
1
1
NTT Basic Research Laboratories, NTT Corporation, Atsugi-shi, Japan
2
NTT Nanophotonics Center, NTT Corporation, Atsugi-shi, Japan
3
Hokkaido University, Sapporo, Japan
Keywords: Er, Sc
2
O
3
, Si, Molecular Beam Epitaxy.
Abstract: We grew (Er
x
Sc
1-x
)
2
O
3
films on Si(111) as a function of x using the molecular beam epitaxy method. The
films were characterized by synchrotron grazing incidence and normal X-ray diffraction, cross-sectional
transmission electron microscopy, and photoluminescence measurements in spectrum and time domains.
We succeeded in obtaining (Er
x
Sc
1-x
)
2
O
3
films on Si(111) that are strained and exhibit 1.5-m light
emission from Er
3+
ions at 4 K and room temperature. We found that the epitaxial Er-doped Sc
2
O
3
films are
better candidates as a light emitting material than epitaxial layers of Er
2
O
3
on Si(111).
1 INTRODUCTION
Integration of cubic (bixbyite-type) sesquioxides on
a Si platform has high potential to improve the
performance of Si circuits by incorporating new
functionalities as alternative gate oxides and light
emitting materials (Grivas, 2008, Reiner, 2010,
Bradley, 2011, Michael, 2009). Among the oxides,
Sc
2
O
3
is one of the most promising as a host material
of rare-earth ions-doped light emitters on Si
substrates (Grivas, 2008, Ter-Gabrielyan, 2011,
Merkle, 2013). The thermal conductivity of Sc
2
O
3
is
the largest among the bixbyite-type oxides,
including Y
2
O
3
and rare earth oxides, and larger than
that of yttrium aluminium garnet (YAG) crystal. The
high thermal conductivity could enable us to obtain
high-power light emission on Si. In fact, recent
researches have shown that Er-doped Sc
2
O
3
ceramics exhibit lasing at the wavelength of 1.58 m
with high quantum efficiency at low temperatures
(Gheorghe, 2008, Kühn, 2009, Ter-Gabrielyan, 2011,
Merkle, 2013). However, to the best of our
knowledge, growth of Er-doped Sc
2
O
3
has not been
achieved on Si, even though Er ions have been
successfully doped in Sc
2
O
3
single and nano-crystals
(Krsmanovic, 2006, Gün, 2007) and the energy
levels of Er
3+
in Sc
2
O
3
ceramics are well-established
(Trabelsi, 2010). This is mainly due to the large
lattice misfit between Sc
2
O
3
and Si. The misfit
between lattice constant of Sc
2
O
3
and two times that
of Si is about 9%. Fortunately, despite the large
misfit, high-quality Sc
2
O
3
films with misfit
dislocations have been grown on a miscut Si(111) as
well as in the system of Gd
2
O
3
on Si(111) by using
the molecular beam epitaxy (MBE) method (Klenov,
2005, Hong, 2005, Chen, 2005). The defects,
including such interfacial dislocations, however,
should be avoided as much as possible for optical
applications.
In this work, we grew epitaxial Er-doped Sc
2
O
3
films on Si(111) using MBE as a function of Er
concentration and characterized the structural and
optical properties of the epitaxial films by
synchrotron grazing incidence X-ray diffraction
(GIXD), X-ray diffraction (XRD), cross-sectional
transmission electron microscope (XTEM), and
photoluminescence (PL) measurements. We will
show that high-quality Er-doped epitaxial Sc
2
O
3
films that exhibit 1.5-m light emission from Er
3+
ions can be grown on Si(111) by using MBE.
2 EXPERIMENTS
The heteroepitaxial growths of Er-doped Sc
2
O
3
were
performed on Si(111) substrates in an MBE chamber
equipped with reflection high-energy diffraction
(RHEED) apparatus. Sc
2
O
3
and Er
2
O
3
were
175
Omi H., Tawara T., Hozumi T., Kaji R., Adachi S., Gotoh H. and Sogawa T..
Molecular Beam Epitaxy of (ErxSc1-x)2O3 on Si(111) for Active Integrated Optical Devices.
DOI: 10.5220/0004762001750179
In Proceedings of 2nd International Conference on Photonics, Optics and Laser Technology (PHOTOPTICS-2014), pages 175-179
ISBN: 978-989-758-008-6
Copyright
c
2014 SCITEPRESS (Science and Technology Publications, Lda.)