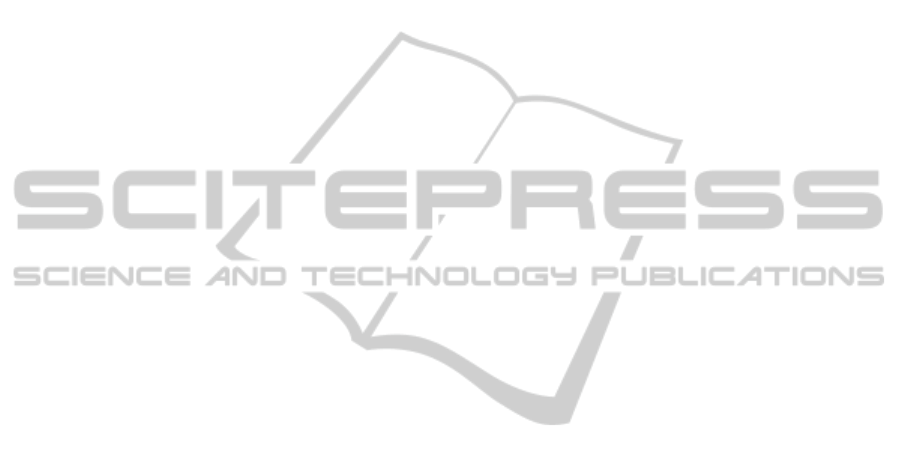
(2007). Indices of cardiovascular function derived
from peripheral pulse wave analysis using radial
applanation tonometry: a measurement repeatability
study. Vascular medicine (London, England), 12(3),
189–97.
Euser, A. M., Dekker, F. W., & le Cessie, S. (2008). A
practical approach to Bland-Altman plots and variation
coefficients for log transformed variables. Journal of
clinical epidemiology, 61(10), 978–82.
Frimodt-Moller, M., Nielsen, A. H., Kamper, A.-L., &
Strandgaard, S. (2008). Reproducibility of pulse-wave
analysis and pulse-wave velocity determination in
chronic kidney disease. Nephrology, dialysis,
transplantation : official publication of the European
Dialysis and Transplant Association - European Renal
Association, 23(2), 594–600.
Kara, S., Okandan, M., Usta, G., & Tezcaner, T. (2004).
Investigation of a new heart contractility power
parameter. Computer methods and programs in
biomedicine, 76(2), 177–80.
Kelly, R., & Fitchett, D. (1992). Noninvasive
determination of aortic input impedance and external
left ventricular power output: a validation and
repeatability study of a new technique. Journal of the
American College of Cardiology, 20(4), 952–963.
Korpas, D., Hálek, J., & Dolezal, L. (2009). Parameters
describing the pulse wave. Physiological research /
Academia Scientiarum Bohemoslovaca, 58(4), 473–9.
Laurent, S., Cockcroft, J., Van Bortel, L., Boutouyrie, P.,
Giannattasio, C., Hayoz, D., … Struijker-Boudier, H.
(2006). Expert consensus document on arterial
stiffness: methodological issues and clinical
applications. European heart journal, 27(21), 2588–
605.
Miller, R. S., Rudra, C. B., & Williams, M. a. (2007).
First-trimester mean arterial pressure and risk of
preeclampsia. American journal of hypertension,
20(5), 573–8.
Pereira, T, Cabeleira, M., Matos, P., Borges, E., Cardoso,
J., & Correia, C. (2011a). Optical Methods for Local
Pulse Wave Velocity Assessment. In 4th International
Joint Conference on Biomedical Engineering Systems
and Technologies Rome Italy (pp. 74–81).
Pereira, T, Santos, I., Oliveira, T., Vaz, P., Santos, H.,
Pereira, H., Cardoso, J. (2013a). Local PWV and other
Hemodynamic Parameters Assessment : Validation of
a New Optical Technique in an Healthy Population. In
6th International Joint Conference on Biomedical
Engineering Systems and Technologies, Barcelona
Spain (Vol. 1, p. 10).
Pereira, Tânia, Oliveira, T., Cabeleira, M., Matos, P.,
Pereira, H. C., Almeida, V., … Correia, C. (2011b).
Signal Analysis in a New Optical Pulse Waveform
Profiler for Cardiovascular Applications. Signal and
Image Processing and Applications / 716: Artificial
Intelligence and Soft Computing, (Sipa), 19–25.
Pereira, Tânia, Santos, I., Oliveira, T., Vaz, P., Correia, T.,
Pereira, T., Correia, C. (2013b). Characterization of
Optical System for Hemodynamic Multi-Parameter
Assessment. Cardiovascular Engineering and
Technology, 4(1), 87–97.
Protogerou, A. D., Papaioannou, T. G., Sfikakis, P. P.,
Blacher, J., Karatzis, E., Lekakis, J. P., … Safar, M. E.
(2012). Differences in pulse pressure day variability
between the brachial artery and the aorta in healthy
subjects. Artery Research, 6(1), 34–40.
Tânia Pereira, Manuel Cabeleira, Patrícia Matos, Elisabeth
Borges, Vânia Almeida, João Cardoso, C. C. (2012).
Non-contact Pulse Wave Velocity Assessment.
Springer-Verlag Berlin Heidelberg 2012, BIOSTEC
2011, CCIS 273, (2), 246–257.
Vanmolkot, F. H. M., & de Hoon, J. N. J. M. (2005).
Reproducibility of forearm vasodilator response to
intra-arterial infusion of calcitonin gene-related
peptide assessed by venous occlusion
plethysmography. British journal of clinical
pharmacology, 59(4), 387–97.
Vappou, J., Luo, J., Okajima, K., Di Tullio, M., &
Konofagou, E. E. (2011). Non-invasive measurement
of local pulse pressure by pulse wave-based ultrasound
manometry (PWUM). Physiological measurement,
32(10), 1653–62.
BIOSIGNALS2014-InternationalConferenceonBio-inspiredSystemsandSignalProcessing
228