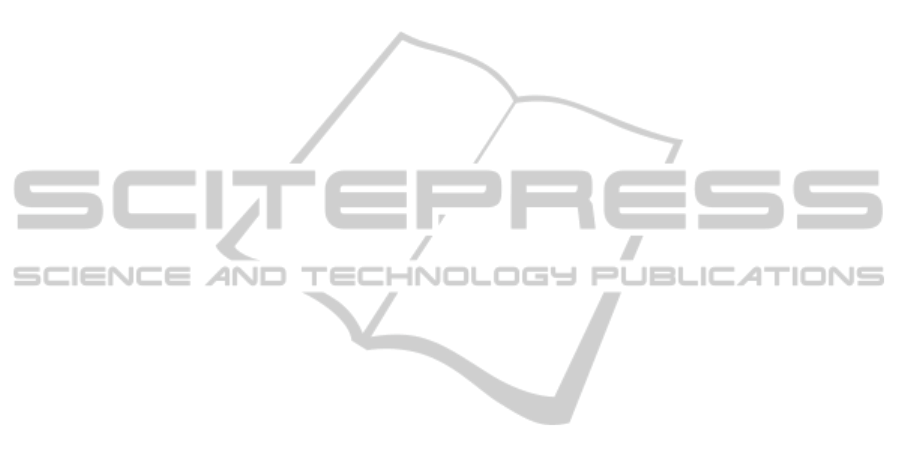
already prepared not only to connect sensors, but
also actuators to perform defined actions for a
closed-loop control strategy.
5 CONCLUSIONS
A telemetric biofeedback concept for continuous
monitoring of bone healing was introduced. The
system allows for autonomous long-term data
collection over several months, carrying a high
potential to significantly improve fracture care.
Feasibility of the approach was proven in an animal
experiment. Collection of further in-vivo data - also
in humans - is the consequent next step.
ACKNOWLEDGEMENTS
The authors would like to thank N. Ramagnano for
his valuable contribution on electronics layout and
development.
This work was performed with the assistance of the
AO Foundation via the AOTRAUMA Network
(Grant No.: AR2012_02).
REFERENCES
Bergmann, G., Deuretzbacher, G., Heller, M., Graichen,
F., Rohlmann, A., Strauss, J., & Duda, G.N. 2001. Hip
contact forces and gait patterns from routine activities.
J.Biomech., 34, (7) 859-871 available from:
PM:11410170.
Bishop, N. E., van, R. M., Tami, I., Corveleijn, R.,
Schneider, E., & Ito, K. 2006. Shear does not
necessarily inhibit bone healing. Clin.Orthop.Relat
Res., 443, 307-314 available from: PM:16462456.
Burny, F., Burny, W., Donkerwolcke, M., & Behrens, M.
2012. Effect of callus development on the deformation
of external fixation frames. Int.Orthop., 36, (12) 2577-
2580 available from: PM:23073925.
Claes, L., Augat, P., Schorlemmer, S., Konrads, C.,
Ignatius, A., & Ehrnthaller, C. 2008. Temporary
distraction and compression of a diaphyseal osteotomy
accelerates bone healing. J.Orthop.Res., 26, (6) 772-
777 available from: PM:18240329.
Claes, L., Grass, R., Schmickal, T., Kisse, B., Eggers, C.,
Gerngross, H., Mutschler, W., Arand, M.,
Wintermeyer, T., & Wentzensen, A. 2002. Monitoring
and healing analysis of 100 tibial shaft fractures.
Langenbecks Arch.Surg., 387, (3-4) 146-152 available
from: PM:12172859.
Claes, L. E., Wilke, H. J., Augat, P., Rubenacker, S., &
Margevicius, K.J. 1995. Effect of dynamization on gap
healing of diaphyseal fractures under external fixation.
Clin.Biomech.(Bristol., Avon.), 10, (5) 227-234
available from: PM:11415558.
Cunningham, J. L., Evans, M., Harris, J. D., & Kenwright,
J. 1987. The measurement of stiffness of fractures
treated with external fixation. Eng Med., 16, (4) 229-
232 available from: PM:3691938.
Eastaugh-Waring, S. J., Joslin, C. C., Hardy, J. R., &
Cunningham, J. L. 2009. Quantification of fracture
healing from radiographs using the maximum callus
index. Clin.Orthop.Relat Res., 467, (8) 1986-1991
available from: PM:19283438.
Epari, D. R., Kassi, J. P., Schell, H., & Duda, G. N. 2007.
Timely fracture-healing requires optimization of axial
fixation stability. J.Bone Joint Surg.Am., 89, (7) 1575-
1585 available from: PM:17606797.
Epari, D. R., Wehner, T., Ignatius, A., Schuetz, M. A., &
Claes, L. E. 2013. A case for optimising fracture
healing through inverse dynamization.
Med.Hypotheses, 81, (2) 225-227 available from:
PM:23688741.
Evans, M., Kenwright, J., & Cunningham, J. L. 1988.
Design and performance of a fracture monitoring
transducer. J.Biomed.Eng, 10, (1) 64-69 available
from: PM:3347037.
Goodship, A. E. & Kenwright, J. 1985. The influence of
induced micromovement upon the healing of
experimental tibial fractures. J.Bone Joint Surg.Br.,
67, (4) 650-655 available from: PM:4030869.
Hente, R., Lechner, J., Fuechtmeier, B., Schlegel, U., &
Perren, S. 2001. Der Einfluss einer zeitlich limitierten
kontrollierten Bewegung auf die Frakturheilung. Hefte
Unfallchirurg (283) 23-24.
McClelland, D., Thomas, P. B., Bancroft, G., &
Moorcraft, C.I. 2007. Fracture healing assessment
comparing stiffness measurements using radiographs.
Clin.Orthop.Relat Res., 457, 214-219 available from:
PM:17159575.
Perren, S. M. 1979. Physical and biological aspects of
fracture healing with special reference to internal
fixation. Clin.Orthop.Relat Res. (138) 175-196
available from: PM:376198.
Seide, K., Aljudaibi, M., Weinrich, N., Kowald, B.,
Jurgens, C., Muller, J., & Faschingbauer, M. 2012.
Telemetric assessment of bone healing with an
instrumented internal fixator: a preliminary study.
J.Bone Joint Surg.Br., 94, (3) 398-404 available from:
PM:22371550.
Wilson, D. J., Morgan, R. L., Hesselden, K. L., Dodd, J.
R., Janna, S. W., & Fagan, M. J. 2009. A single-
channel telemetric intramedullary nail for in vivo
measurement of fracture healing. J.Orthop.Trauma,
23, (10) 702-709 available from: PM:19858978.
BIODEVICES2014-InternationalConferenceonBiomedicalElectronicsandDevices
248