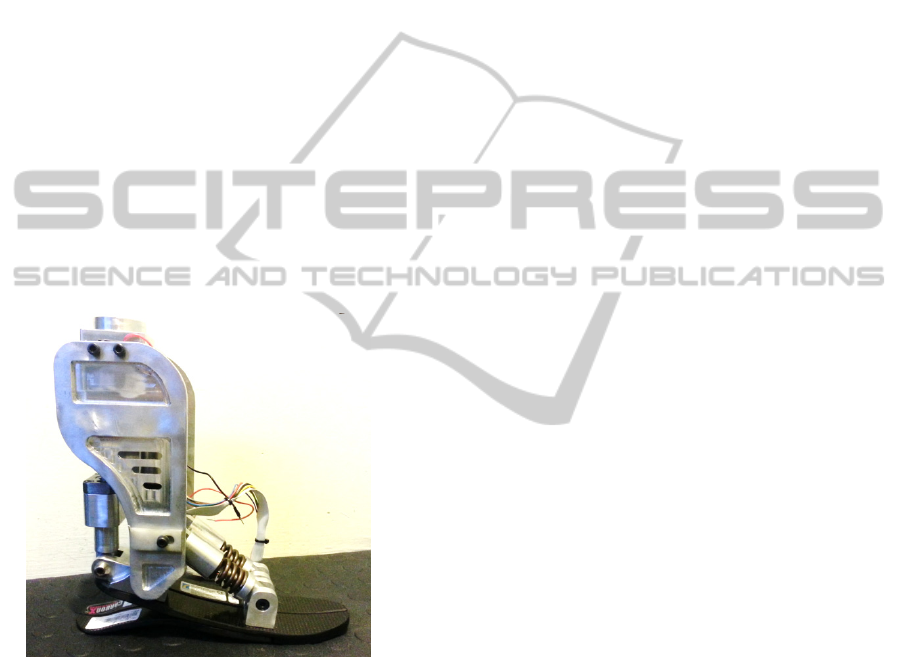
of ensure that the device can withstand dynamic
loads for subjects with a weight less than 85 kg.
These studies were not addressed in this paper. The
mechanism of the ankle module has demonstrated to
provide a full range of motion of 40 degrees, more
than necessary for represent a normal walking. On
the other hand the ankle module is capable to
generate a torque of 135 Nm, enough to mimic the
biological ankle torque at level-ground walking. The
springs that are attached in parallel with the actuator
on the ankle module can be replaced by composite
materials and this change will permit reduce the
overall weight of the ankle module.
The addition of elastic elements and a clutch
mechanism to our design has been demonstrated
theoretically to reduce significantly the electrical
energy requirements required by the motor.
Simultaneously we amplified the force bandwidth of
the actuator. Thus it was permitted that the
prosthesis performs more complex tasks where we
need more power on the actuation, such as walking
upstairs and down stairs, as well as standing from a
seated posture.
Figure 5: Physical prototype of the powered ankle module.
4 CONCLUSIONS
In this paper we describe the design of a prototype
of a powered ankle- knee prosthesis that is human-
like in weight, size and functionality. The
architecture that comprises this prosthetic device
permits to mimic the behaviour of human leg in
normal walking. Consequently it would be capable
to satisfy the necessary requirements such as, torque
and movements range, performing these tasks with
economical electrical-energy consumption. The
prototype of the ankle module is fully assembled as
can be seen in Figure 5. The weight and the size of
the ankle module can be reduced optimizing the
design and replace some elements that it would
permit enhance the torque bandwidth. The knee
module is on manufacturing process, once
completed this process it will start to test the control
system algorithm that will control the actuation of
the prosthesis
ACKNOWLEDGEMENTS
We would like to thanks to sponsors of Bio-
Prosthesis project UABC and Idalia Martinez. Thank
God for giving us strength and knowledge to make
this project.
REFERENCES
A. M. Dollar and H. Herr., 2008. Design of a quassi-
passive knee exoskeleton to assist running, IEEE/RSJ
International Conference on Intelligent Robots and
System, Nice, France.
Au, S. K., Weber, J., and Herr, H., 2009. Powered Ankle-
Foot Prosthesis Improves Walking Metabolic
Economy, Robotics, IEEE Transactions. 25, (1), pp.
51-66.
A. Zoss, H. Kazerooni, and A. Chu, 2005. On the
Mechanical Design of the Berkeley Lower Extremety
Exoskeleton (BLEEX), IEEE/RSJ International
Conference on Intelligent Robots and System.
D. A. Winter, 1991. The Biomechanics and motor control
of Human gait: Normal, elderly and pathological,
Waterloo Biomechanics, vol. 2.
E. J. Rouse, L. M. Mooney, E. C. Martinez-Villalpando
and H. M. Herr, 2013. A clutchable series-elastic
actuator: design of a robotic knee prosthesis for
minimum energy consumption. IEEE International
Conference on Rehabilitation Robotics.
E. Martinez-Villalpando, and Herr, H. M. Herr., 2009.
Agonist-antagonist active knee prosthesis: A
preliminary study in level-ground walking. Journal of
Rehabilitacion Research & Development. Vol.46, no.
3, pp. 361-73.
F. Sup, H. Varol, J. Mitchell, T. Withrow, and M.
Goldfarb, 2009. Self-contained powered knee and
ankle prosthesis: Initial evaluation on a transfemoral
amputee, Rehabilitation Robotics, ICORR, IEEE
International Conference. Pp. 638-644.
G. R. Colborne, S. Naumann, P.E. Longmuir, and D.
Berbrayer, 1992. Analysis of mechanical and
metabolic factors in the gait of congenital below knee
amputees: A comparsion of the SACH and Seattle
feet, Ammer. J. Phys. Med. Rehabil.,vol. 71 no. 5, pp.
272-278.
ALowCostDesignofPoweredAnkle-KneeProsthesisforLowerLimbAmputees-PreliminaryResults
257