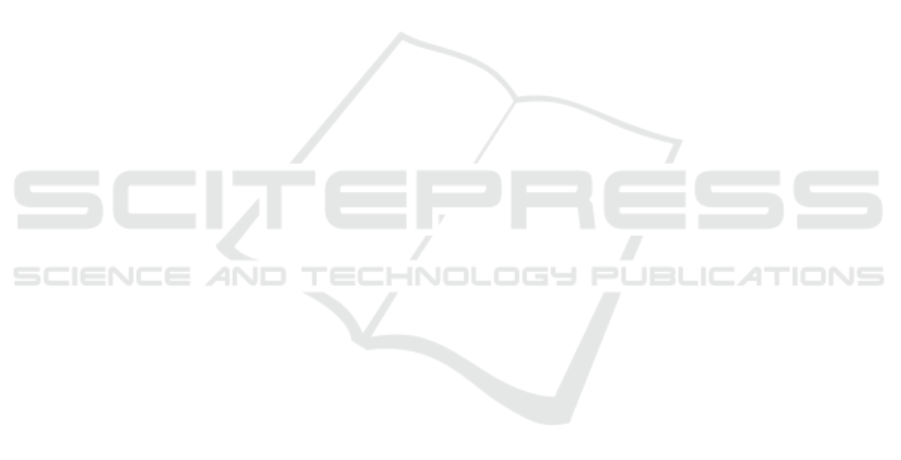
A New Integration Method for Mounting and in vivo
Handling of Sub-mm Flexible Cuff Electrode
Fábio Rodrigues
1,2
and Paulo Mendes
1
1
Centro Algoritmi, University of Minho, Guimarães, Portugal
2
DIMES/ECTM, Delft University of Technology, Delft, The Netherlands
Keywords: Neural Stimulation, Cuff Electrode, Mounting, Polyimide, PDMS.
Abstract: Electrical stimulation of peripheral nerves is commonly used in both research and clinical fields. Cuff
electrodes are one possible interface between nerves and the stimulation microsystem. Among cuff
electrodes, flexible, polyimide-based devices have been demonstrating good and consistent results for the
past 15 years. Regarding mounting and mechanical stability of flexible electrodes for in vivo trials,
improvements are still due. A new concept aiming at the integration of polyimide-based devices in an elastic
handling structure was developed. By taking advantage of PDMS elasticity and moulding, this new
integration method provides surgeons with the ability to move and to rotate the cuff minimizing nerve-
electrode contact and, consequently, nerve damages. A 9 parts stainless steel mould was designed and
fabricated to allow integration of polyimide electrode arrays together with the PDMS mounting structure
and a print circuit board. Furthermore, with the fabricated mould it is possible to achieve a final cylindrical
channel with diameter of 800 µm, as well as handling strips to open and close the cuff.
1 INTRODUCTION
Electrodes are a key component in neural
engineering applications, being used as both
actuators and sensors, i.e. to excite neural tissue by
electrical stimulation or to record bioelectrical
signals from it. Therefore, implantable electrodes
work as interfaces between neural prostheses and the
biological tissue. Among others, potential
applications of implantable electrodes for neural
electrical stimulation range from restoration of
walking (Gustafson et al., 2010) to vagal nerve
stimulation (e.g., epilepsy treatment or heart rate
control) (Connor et al, 2012)and bladder
management in incontinence (Rijkhoff et al, 1997).
Geometrical configuration and dimensions of
electrodes are strongly dependent on the size and
morphology of target nerves. For cylindrical shaped
nerves (e.g., sciatic nerve, vagus nerve) electrodes
made of flexible substrates are a good solution
because they can be wrapped around the nerve.
Usually, this is made by means of cuff electrodes
(Veraart et al, 1993). Cuff electrode diameters range
from hundreds of micrometers (Rodrigues et al,
2012) to few milimeters. During the last two
decades, improvements in the variety and in the
complexity of electrodes for neural stimulation have
provided new solutions in new applications fields
(e.g., vagus nerve stimulation in epislepsy treatment
(Englot et al., 2011, or the FINE electrode for
walking restoration (Schiefer et al., 2008). Many of
these clinical improvements were enabled through
the development of micromachining technologies.
Polyimide-based electrodes have been
demonstrating good and consistent results for the
past 15 years. Polyimides have become an important
and widespread used material for flexible electrodes
due to its good adhesion to metals, long lifetime and
low rate of water retention. Electrodes made of
polyimide usually consist of a thin-film
metallization sandwiched between two layers of
polymer. Thin, uniform layers of polymer are
achieved by means of pouring polyimide on the
substrate followed by spinning. Therefore, the total
thickness of a flexible, polyimide-based electrode is
lower than 30 μm and in vivo handling conditions
require a protective layer between the polyimide and
the biological surrounding tissues.
Biomedical microsystems must fulfil different
requirements for acute and chronic implantation.
When using cuff electrodes for electrical stimulation
of peripheral nerves, three of these requirements are:
265
Rodrigues F. and Mendes P..
A New Integration Method for Mounting and in vivo Handling of Sub-mm Flexible Cuff Electrode.
DOI: 10.5220/0004914602650270
In Proceedings of the International Conference on Biomedical Electronics and Devices (BIODEVICES-2014), pages 265-270
ISBN: 978-989-758-013-0
Copyright
c
2014 SCITEPRESS (Science and Technology Publications, Lda.)