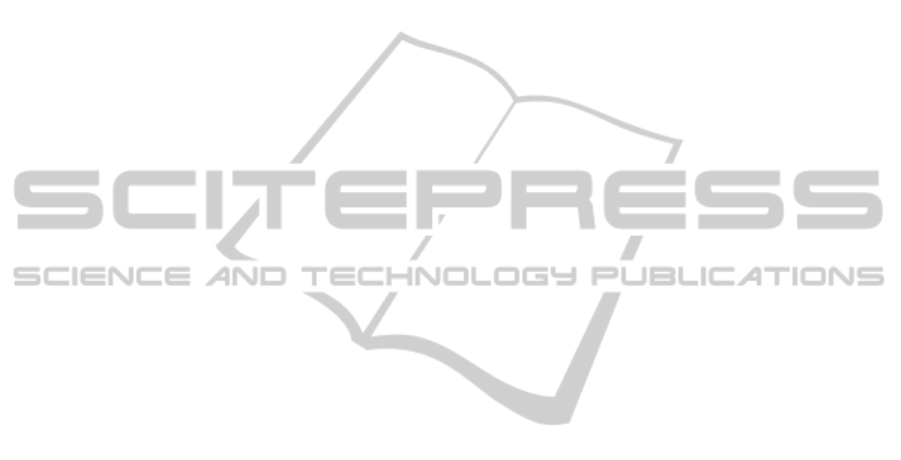
residents and also on energy loss of the building and
therefore costs for the residents, which are not
investigated yet. The problem statement for this
paper is therefore: what are the effects on thermal
comfort if energy is stored in the floor heating
system, what is the energy loss and what are the
related financial consequences?
Contribution of this paper is to develop
mathematical relations and to demonstrate the
relation between FHTES, thermal comfort, energy
loss and energy costs. These insights and relations
are to be used for developing algorithms to control
district heating and heat pumps.
Outline of this paper: Chapter 2 describes related
works on Thermal Activated Building Systems
(TABS), control and performance. Chapter 3 gives
theoretical backgrounds on modelling and thermal
comfort. Chapter 4 describes a case study, followed
by results and discussion in chapter 5. Conclusions
are drawn in chapter 6. The appendix contains a list
of abbreviations and the applied thermal network
model.
2 RELATED WORK
In this section we discuss related work on TABS and
power balancing by FHTES.
FHTES is investigated for different applications.
One of the applications is cooling offices by thermal
activation of floors during night hours (Fellin and
Sommer, 2003), (Lehmann et al., 2007), (Pavlov and
Olesen, 2011), (Rijksen et al., 2010) and (Saelens et
al., 2011). Another application is lowering peak
heating power for residential buildings (Airaksinen
and Vuolle, 2013). More close to our application is a
study on measured performance of heating and
cooling systems using TABS in apartments (Alvi
and Qureshi).
Besides effects on thermal comfort, FHTES also
influences energy loss of the building and therefore
energy costs paid by the residents. In (Scheepens,
2013) the ecocost approach was applied to
investigate a novel house heating/cooling system
with individual room temperature control for the
Meppel project. In (Tahersima et al., 2011) the
contribution of a control strategy for power
balancing and FHTES is investigated. Thermal
tolerance is introduced as a measure for effects on
thermal comfort due to FHTES. In (Verhelst et al.,
2012) optimal heat pump control in case of floor
heating in relation with varying electricity prices is
investigated. Thermal discomfort is introduced as a
measure for effects on thermal comfort. Instead of
using thermal tolerance or thermal discomfort, we
propose in this paper to develop measures from
governing standards on thermal comfort, as outlined
in section 3.2.
Most of the mathematical work within the cited
papers is applicable for general use. However,
effects on thermal comfort, energy loss and energy
costs are not investigated yet in a way corresponding
with thermal comfort standards. Therefore we
investigate how thermal comfort is influenced by
FHTES and from this we deduce constraints and
guidelines for control algorithms.
3 MODELLING APPROACH
In this section we define a simulation model and
constraints from theory on thermal comfort.
3.1 Thermal Network Model
The thermal network approach is a convenient way
to demonstrate the relation between FHTES, energy
demand and effects on thermal comfort. Another
reason to adopt this approach is to use the model
equations for model predictive control within the
TRIANA smart grid control method (Molderink,
2011), which is a part of future work. Accuracy of
thermal networks is demonstrated in (Liu et al.,
2011) and (Bacher and Madsen, 2011).
The applied thermal network for the living room
of typical Dutch low energy house as defined by
(AgentschapNL, 2013) and model equations are
shown in the appendix. The concrete floor, the zone
including internal separation walls, inner and outer
parts of envelope walls and ceiling are modelled as
temperature nodes with a thermal capacitance. Solar
gains are defined at the outer wall node and the zone
node by window transmittance. Heat loss due to
ventilation and infiltration is defined at the zone
node, as well as appliances and people gains.
Heating input is defined at the floor node.
Weather data from Hoogeveen (close to Meppel)
of ambient temperature and global transmittance, i.e.
total solar radiation on the horizontal plane is used
as input data within the model. Solar radiation on
building planes is calculated using correlations by
(Erbs, 1982) and equations by (Duffie, 1980).
Transmitted radiation through the windows is for
25% absorbed by the floor and 75% by the zone
structure. The house orientation is ideal for passive
solar gains and there are no obstacles which could
cast shadows on the house surfaces. In practise, this
may not always be the case, especially not in mid-
SMARTGREENS2014-3rdInternationalConferenceonSmartGridsandGreenITSystems
44