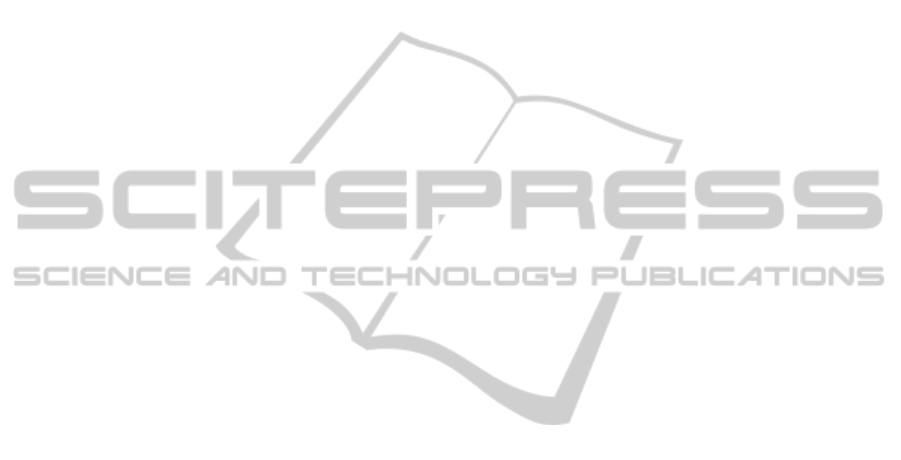
and numerous volatile organic compounds (VOCs)
(Di Francesco et al., 2005); (D’Amico et al., 2007).
The type and number of VOCs in the breath changes
among different individuals, but there is a common
core of breath VOCs which are present in all
individuals. The molecules in an individual’s breath
may be exogenous or endogenous. Exogenous
molecules are those that have inhaled or ingested
from the environment or other sources such as air or
food, thus giving no diagnostic value. Endogenous
molecules are produced by metabolic processes and
partition from blood via the alveolar pulmonary
membrane into the alveolar air. Such endogenous
molecules are present in breath relatively to their
types, concentrations, volatilities, lipid solubility and
rates of diffusion as they circulate in the blood and
cross the alveolar membrane. Changes in the
concentration of the molecules in VOCs could
suggest various diseases or at least changes in the
metabolism.
In this paper, for the first time we present the
design of a portable device able to operate with a
limited number of breath VOCs, normally connected
to oxidative stress, thus giving information to
physicians on the possible state of wellness of an
individual.
2 BREATH ANALYSIS AND
WELLNESS STATE
In this section, we describe the correlation between
exhaled VOCs and oxidative stress. Oxidative stress
is the cause of over 100 diseases, including
atherosclerosis or hypertension. In addition,
hypertension is a risk factor that can lead to
myocardial injury, cardiac failure, aortic aneurysm.
The design of a portable device for breath analysis
must necessarily work on a limited space of breath
VOCs. The most relevant VOCs and their
connection to oxidative stress are labelled as
follows.
Carbon Monoxide (CO): it is naturally produced
by the action of heme oxygenase on the heme for
haemoglobin breakdown. This produces
carboxyhemoglobin, which is a more stable
molecule than oxyhemoglobin. For example, an
increase of CO leads haemoglobin to carry less
oxygen through the vessels. CO is present in
cigarette smoke, and air pollution too. In addition, it
is a blood vessel relaxant (vasodilator), and a
promoter of neurovascular growth, therefore it could
be considered as an indicator of brain tumor growth.
Carbon Dioxide (CO
2
): the variation rate of CO
2
can be associated to different metabolic response,
for example, CO
2
increases during physical activity
or in general with the increment of blood-heat as in a
fever. Analogously, there is a decrease in the case of
hypothermia. In healthy individuals, partial pressure
in arterial blood is very close to the partial pressure
in expired gases. In most forms of lung diseases and
some of congenital heart disease (cyanotic lesions-
bluish-grey discoloration of the skin, lack of O
2
in
the body), a decrease of CO
2
exhaled rate is
commonly observed. Capnography is a technique
commonly used for monitoring the concentration of
CO
2
, or, similarly, its partial pressure using such
technique, the max value of a capnogram
corresponds to the end of tidal volume of exhaled
breath and the steady-state concentrations of each
breath. It must be noted that the breathing rate
influences the level of CO
2
in the blood: slow
breathing rates cause Respiratory Acidosis (i.e.,
increase of blood CO
2
partial pressure, that may
stimulate hypertension, or heart rate acceleration).
On the contrary, too rapid breathing rate leads to
hyperventilation, which may provoke Respiratory
Alkalosis (i.e., decrease of blood CO
2
partial
pressure, no longer fits its role of vasodilator,
leading to possible arrhythmia or heart trouble).
Ethanol: the ethanol compound in a breath
composition can be classified as endogenous or
exogenous. Ethanol endogenous may increase in
exhaled gas mixtures because of alcoholic
fermentation of an excessive over-load of
carbohydrates. Indeed it is originated from microbial
fermentation of carbohydrates in the gastro-intestinal
tract. Then, it enters in the blood. On the contrary,
Ethanol exogenous comes from alcoholic drinks. It
is important to note that it is recognized that ethanol
breakdown leads to an accumulation of free radicals
into the cells, a clear example of oxidative stress.
Ethanol may cause arrhythmias and depresses the
contractility of cardiac muscle.
Nitrogen Oxide (NO): it is a vasodilator and it
modulates inflammatory response (operating in
combination with CO and Hydrogen Sulfide).
Hydrogen Sulfide (H
2
S): it is a vascular relaxant
agent, and has a therapeutic effect in various
cardiovascular diseases (myocardial injury,
hypertension). In general, H
2
S could have
therapeutic effect against oxidative stress due to its
capability to neutralize the action of free radicals. In
patients with coronary heart disease, H
2
S level in
blood is normally reduced, as in the case of
hypertension. On the contrary, in haemorrhagic
shock, H
2
S plasma levels are increased.
Ammonia (NH
3
): an increase of NH
3
in blood
HEALTHINF2014-InternationalConferenceonHealthInformatics
578