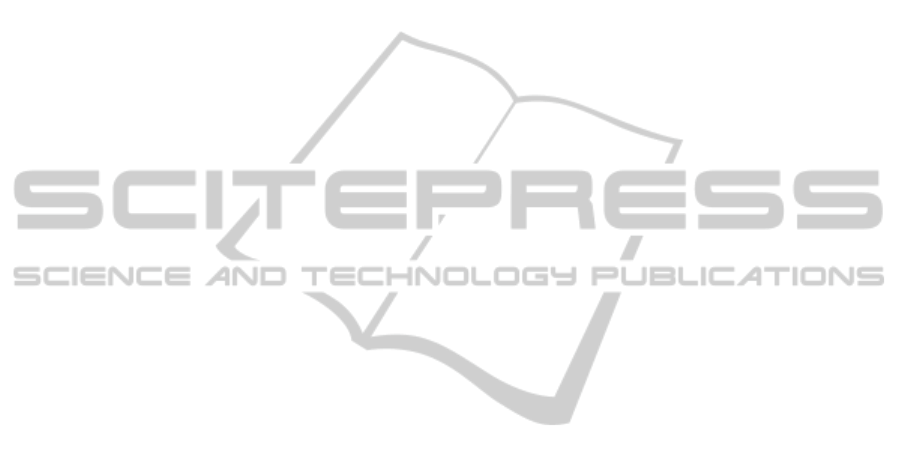
(several mW) to achieve the same flow rate level
compared to the original electrolytic pump, which
makes the integration of wireless power transfer
techniques (Yi et al., 2013) and drug delivery
systems feasible.
4 CONCLUSIONS
This work presents a prototype electrolytic pump
that uses a Pt coated-nickel mesh in the pumping
chamber to improve the cycling time of an
electrolytic pump intended for a drug delivery
system. Using our catalytic reforming element, the
cyclical actuation of the drug delivery system
improved both in terms of the time of the
pump/recombination cycle, as well as the applied
power requirement, resulting in a faster and more
efficient drug delivery system.
REFERENCES
Nisar, A., Afzulpurkar, N., Mahaisavariya, B., &
Tuantranont, A. (2008). MEMS-based micropumps in
drug delivery and biomedical applications. Sensors
and Actuators B: Chemical, 130(2), 917-942.
Teymoori, M. M., & Abbaspour-Sani, E. (2005). Design
and simulation of a novel electrostatic peristaltic
micromachined pump for drug delivery applications.
Sensors and Actuators A: Physical, 117(2), 222-229.
Junwu, K., Zhigang, Y., Taijiang, P., Guangming, C., &
Boda, W. (2005). Design and test of a high-
performance piezoelectric micropump for drug
delivery. Sensors and Actuators A: Physical, 121(1),
156-161.
Hwang, S. R., Sim, W. Y., Kim, G. Y., Yang, S. S., &
Pak, J. J. (2005, May). Fabrication and test of a
submicroliter-level thermopneumatic micropump for
transdermal drug delivery. In Microtechnology in
Medicine and Biology, 2005. 3rd IEEE/EMBS Special
Topic Conference on (pp. 143-145). IEEE.
Guo, S., & Fukuda, T. (2004, April). SMA actuator-based
novel type of micropump for biomedical application.
In Robotics and Automation, 2004. Proceedings.
ICRA'04. 2004 IEEE International Conference on
(Vol. 2, pp. 1616-1621). IEEE.
Su, Y. C., & Lin, L. (2004). A water-powered micro drug
delivery system. Microelectromechanical Systems,
Journal of, 13(1), 75-82.
Li, P. Y., Shih, J., Lo, R., Saati, S., Agrawal, R.,
Humayun, M. S., ... & Meng, E. (2008). An
electrochemical intraocular drug delivery device.
Sensors and Actuators A: Physical, 143(1), 41-48.
Li, P. Y., Sheybani, R., Gutierrez, C. A., Kuo, J. T., &
Meng, E. (2010). A Parylene bellows electrochemical
actuator. Microelectromechanical Systems, Journal of,
19(1), 215-228.
Sheybani, R., & Meng, E. (2012). High-Efficiency MEMS
Electrochemical Actuators and Electrochemical
Impedance Spectroscopy Characterization.
Pirmoradi, F. N., Jackson, J. K., Burt, H. M., & Chiao, M.
(2011). A magnetically controlled MEMS device for
drug delivery: design, fabrication, and testing. Lab on
a Chip, 11(18), 3072-3080.
Yi, Y., Buttner, U., & Foulds, I. G. (2013, October).
TOWARDS AN IMPLANTABLE PULSED MODE
ELECTROLYTIC DRUG DELIVERY SYSTEM. In
Proc. Micro Total Analysis Systems.
Maruyama, J., Inaba, M., Katakura, K., Ogumi, Z., &
Takehara, Z. I. (1998). Influence of Nafion® film on
the kinetics of anodic hydrogen oxidation. Journal of
Electroanalytical Chemistry, 447(1), 201-209.
Yi, Y., Buttner, U., Fan, Y., & Foulds, I. G. (2013, May).
3-Coil resonance-based wireless power transfer system
for implantable electronic. In Wireless Power Transfer
(WPT), 2013 IEEE (pp. 230-233). IEEE.
BIODEVICES2014-InternationalConferenceonBiomedicalElectronicsandDevices
298