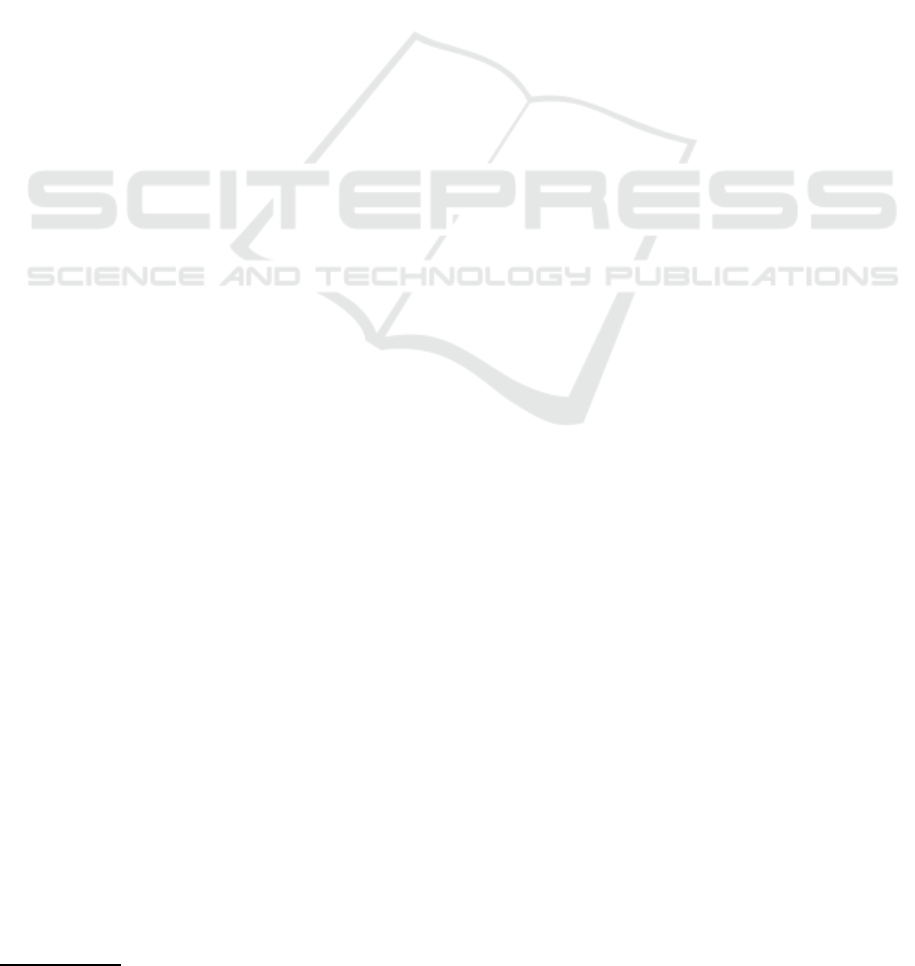
Cooperation Strategies for Multi-user Transmission in Manhattan
Environment
∗
Jaewon Chang and Wonjin Sung
Department of Electronic Engineering, Sogang University, 1 Sinsu-dong, Mapo-gu, 121-742 Seoul, Korea
Keywords:
Cooperative Transmission, MU-MIMO, CoMP, Manhattan Environment.
Abstract:
One of the major drawbacks for wirelesscommunication systems in Manhattan environment with tall buildings
lining both sides of the streets is the performance degradation caused by the penetration loss and the effects of
inter-sector interference. To overcome such a degradation, cooperation among the sectors is under an active
investigation as an efficient means to provide an enhanced coverage as well as increased spectral efficiency. In
this paper, we describe various type of cooperation sector location for cooperative multi-user transmission, and
determine cooperation strategies for the cooperative operation among sectors by evaluating and comparing the
types of microcell in Manhattan environment. The results shows that the more suitable number of cooperation
sectors is determined by signal-to-interference plus noise ratio (SINR), outage probability, and throughput
comparison. Performance variations based on different numbers of sector density under cooperation are also
presented to suggest an efficient inter-sector cooperative transmission strategy in Manhattan environment.
1 INTRODUCTION
In wireless communication systems, mobile user’s
demands are rapidly increasing for high data rate
and quality network services in urban areas. Ur-
ban micro-cellular system in Manhattan-like environ-
ment have difference features, compared with macro-
cellular systems. The micro-cellular network requires
higher system capacity for the dense traffic channel,
where a significant amount of access users to wireless
link occurs. The micro-cellular system is developed
in the metropolitan area, where tall buildings are ag-
gregated and lined both sides of the streets. Thus, the
propagation models of line-of-sight (LOS) and non
line-of-sight (NLOS) wireless channel between base-
stations (BSs) and mobile-stations (MSs) are adopted
for practical performance investigations.
The performance evaluation of micro-cellular net-
works in Manhattan-like environment and related
works have been widely studied. Standardization
groups of IEEE802.16m and 3GPP-LTE advanced
are also developing the next generation cellular sys-
tems considering Manhattan environment. Capacity
and interference statistics of street cross-shaped mi-
cro cells is analyzed in (Ahmed, 2003), and spatial
∗
This work wassupported by the grant from the National
Research Foundation of Korea (NRF) funded by the Korean
government (MEST) (no. 2011-0016146).
multiple-input multiple-output (MIMO) channel ca-
pacity statistics in Manhattan environment are repre-
sented (Chizhik, 2003). The efficient resource con-
trol and channel allocation method are also proposed.
Considering user mobility characteristics, the perfor-
mance focused on average number of handoffs has
been analyzed by (Cho, 2000). The propagation
model and deployment strategies of transmitters was
suggested in (Chiu, 2009), and multi-hop relay net-
works in Manhattan-like environment is investigated
in (Fu, 2007). However, these studies are based on the
non-cooperative operation among BSs without con-
sidering the cooperativetransmission and interference
mitigation.
In this paper, we present the efficient coopera-
tion strategy in Manhattan environment from the per-
formance investigation of the cooperative multi-user
transmission. First, the various cooperation strategies
are presented as increasing the number of coopera-
tion sector antennas, where the uniformly distributed
MSs are located along the cross shape street consider-
ing the urban micro-cellular model of IEEE802.16m
system. For the different BS density in this environ-
ment, cooperation scenarios according to the cooper-
ation sector antenna variation are also described. Sec-
ond, the presented cooperation strategies are applied
to cooperative multi-user transmission using zero-
forcing beamforming and their performance in terms
415
Chang J. and Sung W..
Cooperation Strategies for Multi-user Transmission in Manhattan Environment.
DOI: 10.5220/0004970304150419
In Proceedings of the 16th International Conference on Enterprise Information Systems (ICEIS-2014), pages 415-419
ISBN: 978-989-758-028-4
Copyright
c
2014 SCITEPRESS (Science and Technology Publications, Lda.)