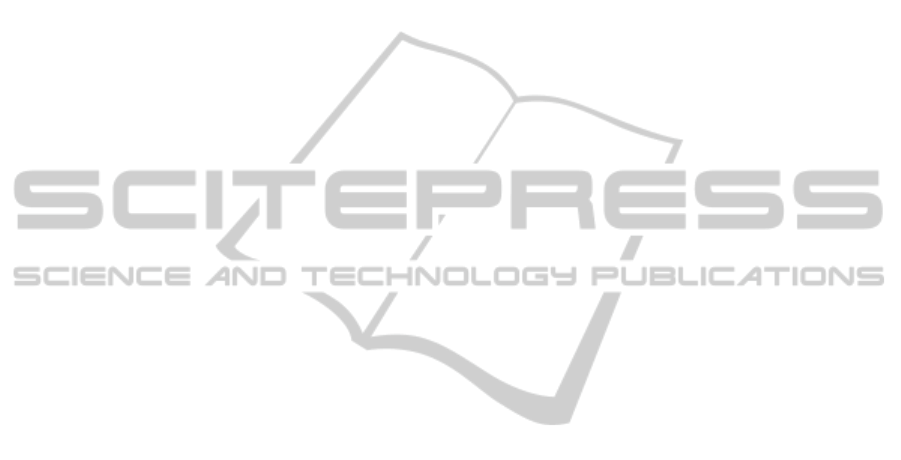
5 CONCLUSIONS
The integrated pyroprocessing features are a batch
type operation, complicated recycling, and tangled
operation logic. An item flow model based on DES
enables a flow control, mass balance calculation, and
basic framework of an integrated pyroprocessing
simulator. Compared to a static or equilibrium
material flow, a model-based dynamic material flow
provides detailed information and thus a careful
analysis of every batch is necessary to confirm the
mass balance results. Verification and validation
regarding the model built thus far has been
performed in terms of the mass balance calculation,
and shows the completeness of the model. However,
the modeling has not been finished but is still under
progress.
To improve the operation model toward a multi-
purpose integrated pyroprocessing simulator, various
modules must be incorporated at a facility level. One
of the issues on a new recycling process such as
pyroprocessing is to guarantee integrated safeguards
in terms of material accountancy and security. Mass
tracking is the most fundamental requirement for a
model to cope with for safeguards assessment. Since
the material flow framework in the current model
can support a perfect mass tracking on an element
basis, a safeguards module is expected to be
developed without difficulty and to be added in an
integrated simulator. Technical feasibility can also
be supported by an integrated simulator to determine
or recommend process operation conditions by
adding an optimization module. Compared to other
reprocessing technologies, economic feasibility must
be tested in a simulation by developing a cost
evaluation module. It is expected that an integrated
pyroprocessing simulator fulfilling the above
described functions by add-on modules will be
released in a few years.
ACKNOWLEDGEMENTS
This work was supported by Nuclear Research and
Development Program of National Research
Foundation of Korea (NRF) funded by Ministry of
Science, ICT and Future Planning (MSIP).
REFERENCES
DePaoli, D., 2011. Modeling and simulation of nuclear
fuel recycling systems, short course of “Introduction to
nuclear chemistry and fuel cycle separations.”
Bechard, V., 2013. Simulation of mixed discrete and
continuous systems: an iron ore terminal example, In
Proceeding of the 2013 Winter Simulation Conference,
1167-1178.
ExtendSim Simulation Software. Imagine That Inc, 2014.
Web. 24 Jun 2014. <http://www.extendsim.com>
Lee, H. J. et al., 2012. Pyroprocessing baseline flowsheet
v4.0, talks in KAERI.
Lee, H. J. et al., 2013. Design for integrated
pyroprocessing plant level simulator, Annals of
Nuclear Energy, 60, 316-328.
Phongikarron, S., Herrmann, S., Simpson, M., 2011.
Diffusion model for electrolytic reduction of uranium
oxides in a molten LiCl-Li2O slat, Nuclear
Technology, 174, 85-93.
Yoo, J. H. et al., 2008. A conceptual study of
pyroprocessing for recovering actinides from spent
oxide fuels. Nucl. Eng. Technol. 40, 581–592.
Song, K. C., Lee, H., Hur, J. M., Kim, J. G., Ahn D. H.,
and Cho, Y. J., 2010. Status of pyroprocessing
technology development in Korea, Nuclear
Engineering Technology, 42(2), 131-144.
Lee, H., Park, G. I., Kang, K. H., Hur, J. M., Kim, J. G.,
Ahn, D. H., Cho, Y. J., and Kim, E. H.,
Pyroprocessing Technology Development at KAERI,
Nucl Eng Tech, 43(4), 317-328.
Karell, E. J., and Gourishankar, K. V., 2001. Separation of
Actinides from LWR Spent Fuel Using Molten Salt
Based Electrochemical Process, Nucl. Tech., 136, 342.
S. D. Herrmann, S. X. Li, and M. F. Simpson, 2005.
Electrolytic Reduction of Spent Oxide Fuel – Bench-
Scale Test Results, Proc. Global 2005, No. 488,
Tsukuba, Japan, October 9-October 13.
J. M. Hur, I. K. Choi, S. H. Cho, S. M. Jeong, C. S. Seo,
2008. Preparation and Melting of Uranium from U3O8
J of Alloys and Compounds, 452, 23.
ICINCO2014-11thInternationalConferenceonInformaticsinControl,AutomationandRobotics
596