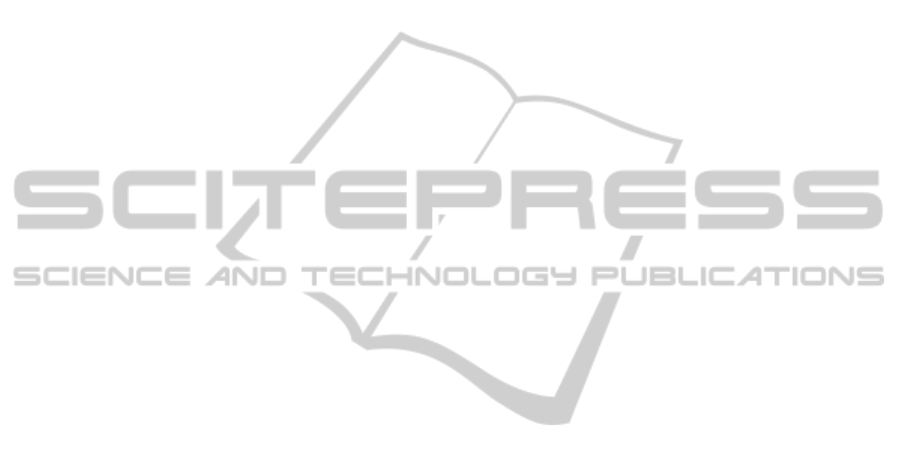
putation of the deformed vibrissa and reconstruction
of the profile as well. Furthermore, an explicit an-
alytical formula to determine the contact point out of
the “measured” values of the observables was derived.
Both will increase the efficiency in experiments in fu-
ture.
These results were obtained without assuming any
estimation or approximation of describing functions.
This is rather new in literature, in contrast to (Kim and
M
¨
oller, 2007), (Birdwell et al., 2007).
Further on, to mimick experimental data, a re-
construction based solely on the “observables” with
added random noise (uncertainty — mimicking noise
in experiments) is valid for various profiles. But, ob-
viously, the contact point approximation accuracy di-
minished from 10
−6
to 10
−2
(dimensionless), i.e., if
the vibrissa is 1 m long then the obstacle contact po-
sition can be determined in the plane with an accu-
racy of 1 cm by a single measuring point during ob-
stacle contour sensing. These results maintain the
hypothesis from biologists, that animals can navigate
by strongly relying on their mechanoreceptors at the
FSC.
Near future (theoretical) work is addressed to the
following investigations:
• analysis of the influence of an elastic support as in
the biological paragon (Behn, 2013a): This could
be needed to guarantee a bounded bending mo-
ment in controlling the support stiffness (i.e., the
vibrissa does not brake during sensing – just think
about a cat passing a fence).
• investigations on non-strictly convex profiles:
There can appear flat points and we have to ad-
just our theory.
• switching from investigations in the vertical x-y-
plane to a 3-dimensional sensing problem.
Intermediate future (experimental) work is addressed
to experiments. At present, we are working on a de-
sign of a prototype for sensing obstacles.
Far future work is addressed to an application of
such tactile sensors to mobile robotics (or a mouse-
like robot) for online object localization and different
tasks similar to the prototypes presented in (Kim and
M
¨
oller, 2006) and (Pearson et al., 2011).
REFERENCES
Abramowitz, M. and Stegun, I. A. (1972). Handbook
of mathematical functions: With formulas, graphs,
and mathematical tables, volume 55 of National Bu-
reau of Standards applied mathematics series. United
States Department of Commerce, Washington, DC,
10. print., dec. 1972, with corr edition.
Behn, C. (2013a). Mathematical Modeling and Control of
Biologically Inspired Uncertain Motion Systems with
Adaptive Features. PhD thesis, Technische Univer-
sit
¨
at Ilmenau, Ilmenau.
Behn, C. (2013b). Modeling the behavior of hair follicle
receptors as technical sensors using adaptive control.
In ICINCO (1), pages 336–345.
Birdwell, J. A., Solomon, J. H., Thajchayapong, M., Taylor,
M. A., Cheely, M., Towal, R. B., Conradt, J., and Hart-
mann, M. J. Z. (2007). Biomechanical Models for Ra-
dial Distance Determination by the Rat Vibrissal Sys-
tem. Journal of Neurophysiology, 98(4):2439–2455.
Clements, T. N. and Rahn, C. D. (2006). Three-dimensional
contact imaging with an actuated whisker. IEEE
Transactions on Robotics, 22(4):844–848.
Hirose, S., Inoue, S., and Yoneda, K. (1989). The whisker
sensor and the transmission of multiple sensor signals.
Advanced Robotics, 4(2):105–117.
Kim, D. and M
¨
oller, R. (2006). Passive sensing and active
sensing of a biomimetic whisker. In Rocha, L. M.,
editor, Artificial life X, A Bradford book, pages 282–
288. MIT Press, Cambridge, Mass.
Kim, D. and M
¨
oller, R. (2007). Biomimetic whiskers for
shape recognition. Robotics and Autonomous Systems,
55(3):229–243.
Pammer, L., O’Connor, D. H., Hires, S. A., Clack, N. G.,
Huber, D., Myers, E. W., and Svoboda, K. (2013).
The mechanical variables underlying object localiza-
tion along the axis of the whisker. The Journal of
neuroscience : the official journal of the Society for
Neuroscience, 33(16):6726–6741.
Pearson, M. J., Mitchinson, B., Sullivan, J. C., Pipe,
A. G., and Prescott, T. J. (2011). Biomimetic vib-
rissal sensing for robots. Philosophical Transac-
tions of the Royal Society B: Biological Sciences,
366(1581):3085–3096.
Prescott, T., Pearson, M., Mitchinson, B., Sullivan, J.
C. W., and Pipe, A. (2009). Whisking with robots:
From Rat Vibrissae to Biomimetic Technology for Ac-
tive Touch. IEEE Robotics & Automation Magazine,
16(3):42–50.
Scholz, G. R. and Rahn, C. D. (2004). Profile Sensing With
an Actuated Whisker. IEEE Transactions on Robotics
and Automation, 20(1):124–127.
Steigenberger, J. (2013). A continuum model of passive
vibrissae.
Tuna, C., Solomon, J. H., Jones, D. L., and Hartmann, M.
J. Z. (2012). Object shape recognition with artificial
whiskers using tomographic reconstruction. In IEEE
International Conference on Acoustics, Speech and
Signal Processing (ICASSP), pages 2537–2540, Pis-
cataway, NJ. IEEE.
Will, C. (2013). Anwendung nichtlinearer Biegetheorie
auf elastische Balken zur Objektabtastung am Beispiel
passiver Vibrissen mit unterschiedlicher Lagerung
(Application of non-linear beam theory for obstacle
detection with respect to the biological paragon of a
passive vibrissa using different supports). Master the-
sis, Technische Universit
¨
at Ilmenau, Ilmenau.
ObjectContourReconstructionusingBio-inspiredSensors
467