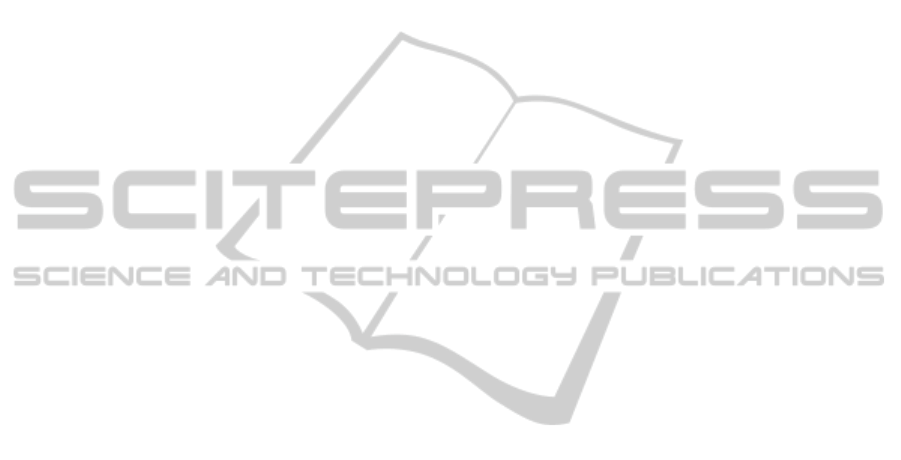
combustion chamber, not yet reached by the
principal flame front, in order to detect the possible
occurrence of knocking through numerical
simulations.
Under proper operating conditions, computations
show a good agreement with experiments as regards
the knocking onset and its temporal location. The
spatial position being the most probable for
knocking is also highlighted. The chemical reactivity
in the zone not yet reached by the flame front
increases as the spark advance is increased, also as a
consequence of the greatest time needed for flame
initiation consequent the lower in-chamber value of
temperature and pressure at spark timing.
According to the performed analysis, a criterion
is established to individuate the knocking occurrence
from the pressure cycle trend.
The developed model is finally included within
an optimization problem aimed at maximizing the
engine power output by proper choice of the
injection strategy and the time of spark ignition with
the avoidance of knocking.
The described simulation approach may be
employed in the phase of engine design to reduce the
time-to-market of new technologies. If reliable
experimental data available for engines of analogous
configurations for validation are missing, the
proposed approach may even furnish qualitative
information useful for the development of control
strategies.
REFERENCES
Alkidas, A. C., 2007. Combustion advancements in
gasoline engines, Energy Conversion and
Management, Vol. 48, pp. 2751–2761.
Allocca, L., Costa, M., Montanaro, A., Sementa, P., Sorge,
U., Vaglieco, B.M., 2012. Characterization of the
Mixture Formation Process in a GDI Engine Operating
in Stratified Mode, 12th Triennial Int. Conf. on Liquid
Atomization and Spray Systems, Heidelberg.
Carling, R. W., 2010. Predictive Simulation of
Combustion Engine Performance in an Evolving Fuel
Environment, Sandia National Laboratories.
Colin, O., Benkenida, A., Angelberger, C., 2003. 3D
Modeling of Mixing, Ignition and Combustion
Phenomena in Highly Stratified Gasoline Engines, Oil
& Gas Science and Technology – Rev. IFP Energies
Nouvelles, Vol. 58, pp. 47-62.
Costa, M., Sorge, U., Allocca, L., 2012. CFD optimization
for GDI spray model tuning and enhancement of
engine performance, Advances in Engineering
Software, Vol. 49, pp. 43-53.
Costa, M., Marchitto, L., Merola, S.S., Sorge, U., 2014.
Study of mixture formation and early flame
development in a research GDI engine through
numerical simulation and UV-digital imaging, Energy,
doi: 10.1016/j.energy.2014.04.114.
Dukowicz, J.K., 1979. Quasi-steady droplet change in the
presence of convection, informal report Los Alamos
Scientific Laboratory, Los Alamos Report LA7997-
MS.
Griffiths, J.F., Hughes, K.J., Schreiber, M., Poppe, C.,
Dryer, F.L., 1994. A unified approach to the reduced
kinetic modeling of alkane combustion, Combustion
and Flame, Vol. 99 (3-4), pp. 533-540.
Halstead, M.P., Kirsch, L.J., Quinn, C.P., 1977. The auto-
ignition of hydrocarbon fuel at high temperatures and
pressures-fitting of a mathematical model, Combustion
and Flame, Vol. 30, pp. 45-60.
Heywood, J.B., 1988. Internal Combustion Engine
Fundamentals, New York: McGraw-Hill.
Huh, K.Y., Gosman, A.D., 1991. A phenomenological
model of diesel spray atomisation, International
Conference on Multiphase Flows, Tsukuba, Japan.
Kuhnke, D., 2004. “Spray Wall Interaction Modeling by
Dimensionless Data Analysis”, PhD thesis,
Technische Universität Darmstadt.
Leppard, W.R, 1991. The autoignition chemistries of
octane-enhancing ethers and cyclic ethers: A motored
engine study, SAE Paper 912313.
Li, H., Prabhu, S., Miller, D., Cernansky, N., 1994.
Autoignition Chemistry Studies on Primary Reference
Fuels in a Motored Engine, SAE Tech. Paper 942062.
Mundo, C., Sommerfeld, M., Tropea, C., 1995. Droplet-
Wall Collisions: Experimental Studies of the
Deformation and Breakup Process, International
Journal of Multiphase Flows, Vol. 21(2), pp. 151-173.
Nelder, J. A., Mead, R., 1965. A simplex method for
function minimization, Computer Journal, Vol. 7, pp.
308–313.
Nordin, W.H., 2001. Complex Modeling of Diesel Spray
Combustion, Thesis (PhD), Chalmers University of
Technology.
Oh H. C., Bae C. S., 2013. Effects of the injection timing
on spray and combustion characteristics in a spray-
guided DISI engine under lean-stratified operation,
Fuel, Vol. 107, pp. 225–235.
O’Rourke, P.J., Bracco, F.V., 1980. Modeling of Drop
Interactions in Thick Sprays and a Comparison with
Experiments, IMECHE, London.
Park, C. Kim, S., Kim, H. Moriyoshi, Y., 2012. Stratified
lean combustion characteristics of a spray-guided
combustion system in a gasoline direct injection
engine, Energy, Vol. 41, pp. 401-407.
Ramos, J. I., 1989. Internal Combustion Engine
Modelling, CRC Press.
Stan, C., 2000. Direct injection systems for spark-ignition
and compression-ignition engines, SAE Publication.
Shi, Y., Ge, H. W., Reitz, R. D., 2011. Computational
Optimization of Internal Combustion Engines,
Springer-Verlag. London.
Thévenin, D., Janiga, G. (Eds.), 2008. Optimization and
Computational Fluid Dynamics, Springer-Verlag.
Berlin Heidelberg.
ModelingandPerformanceOptimizationofaDirectInjectionSparkIgnitionEnginefortheAvoidanceofKnocking
495