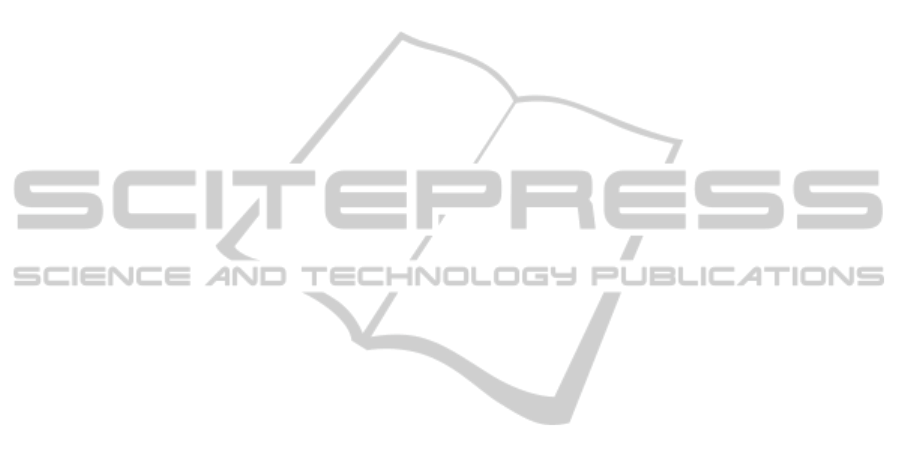
studies of practical application in Indonesia, Thailand
and Japan. Tsunami evacuation feasibility, casualty
estimation, bottleneck identification, shelter alloca-
tion and other applications were modeled to support
tsunami mitigation activities.
ACKNOWLEDGEMENTS
We express our deep appreciation to JST-JICA
SATREPS projects, the Ministry of Education, Cul-
ture, Sports, Science and Technology (MEXT) in
Japan, and the International Research Institute of Dis-
aster Science (IRIDeS) at Tohoku University, Japan
for their support.
REFERENCES
Bernard, E., Mofjeld, H. O., Titov, V. V., Synolakis, C. E.,
and Gonz
´
alez, F. I. (2006). Tsunami: scientific fron-
tiers, mitigation, forecasting and policy implications.
Philosophical transactions of The royal Society. Se-
ries A, Mathematical, physical, and engineering sci-
ences, 364(1845):1989–2007.
Fujioka, M., Ishibashi, K., Kaji, H., and Tsukagoshi, I.
(2002). Multi agent Simulation Model for Evaluat-
ing Evacuation Management System Against Tsunami
Disaster. Journal of Architecture, Planning and Envi-
ronmental Engineering, 562:231–236.
Imamura, F. (1996). Review of tsunami simulation with a
finite difference method. In Yeh, H., Liu, P., and Syno-
lakis, C. E., editors, Long-Wave Runup Models, pages
25–42. World Scientific Publishing Co., Singapore.
Imamura, F., Muhari, A., Mas, E., Pradono, M. H., Sugi-
moto, M., and Post, J. (2012). Tsunami Disaster Mit-
igation by Integrating Comprehensive Countermea-
sures in Padang City, Indonesia. Journal of Disaster
Research, 7(1).
Japan Meteorological Agency (JMA) (2013). Lessons
learned from the tsunami disaster caused by the 2011
Great East Japan Earthquake and improvements in
JMA ’ s tsunami warning system October 2013 Japan
Meteorological Agency. Technical Report October,
Japan Meteorological Agency (JMA).
Kato, S., Shimozono, T., and Okayasu, A. (2009). Hy-
brid Simulation for Tsunami Evacuation in Consider-
ation of Individual Behaviors. Journal of Japan Soci-
ety of Civil Engineers, Ser. B2 (Coastal Engineering),
65(1):1316–1320.
L
¨
ammel, G., Rieser, M., Nagel, K., Taubenb
¨
ock, H., Strunz,
G., Goseberg, N., Schlurmann, T., Kl
¨
upfel, H., Se-
tiadi, N., and Birkmann, J. (2010). Emergency Pre-
paredness in the case of a Tsunami - Evacuation Anal-
ysis and Traffic Optimization for the Indonesian city
of Padang. In Klingsch, W., Rogsch, C., Schad-
schneider, A., and Schreckenberg, M., editors, Pedes-
trian and Evacuation Dynamics 2008, pages 171–182.
Springer, Berling Heidelberg.
Mas, E., Adriano, B., and Koshimura, S. (2013a). An In-
tegrated Simulation of Tsunami Hazard and Human
Evacuation in La Punta , Peru. Journal of Disaster
Research, 8(2):285–295.
Mas, E., Suppasri, A., Imamura, F., and Koshimura, S.
(2012). Agent-based Simulation of the 2011 Great
East Japan Earthquake / Tsunami Evacuation : An In-
tegrated Model of Tsunami Inundation and Evacua-
tion. Journal of Natural Disaster Science, 34(1):41–
57.
Mas, E., Suppasri, A., Srivihok, P., and Koshimura, S.
(2013b). Feasibility of Evacuation at the Pakarang
Cape in Thailand based on Tsunami Inundation Model
and Human Evacuation Simulation. In 10th Interna-
tional Conference on Urban Earthquake Engineering,
pages 1–6, Tokyo, Japan.
Meguro, K. and Oda, K. (2005). Development of Evacua-
tion Simulator for Tsunami Disaster Mitigation. Pro-
duction Research, 57(4):343–347.
Murakami, H., Takimoto, K., and Pomonis, A. (2012).
Tsunami Evacuation Process and Human Loss Distri-
bution in the 2011 Great East Japan Earthquake - A
Case Study of Natori City , Miyagi Prefecture -. In
15th World Conference on Earthquake Engineering,
pages 1–10.
Shuto, N. (2009). Tsunami Research - Its Past, Present
and near Future -. In Proceedings of the Sixth In-
ternational Workshop on Coastal Disaster Prevention,
pages 1–24, Bangkok, Thailand.
Suppasri, A., Shuto, N., Imamura, F., Koshimura, S., Mas,
E., and Yalciner, A. C. (2012). Lessons Learned from
the 2011 Great East Japan Tsunami: Performance
of Tsunami Countermeasures, Coastal Buildings, and
Tsunami Evacuation in Japan. Pure and Applied Geo-
physics, 170(6-8):993–1018.
Suzuki, T. and Imamura, F. (2005). Simulation model of
the evacuation from a tsunami in consideration of the
resident consciousness and behavior. Journal of Japan
Society for Natural Disaster Science, 23(4):521–538.
Synolakis, C. E. and Bernard, E. N. (2006). Tsunami sci-
ence before and beyond Boxing Day 2004. Philosoph-
ical transactions. Series A, Mathematical, physical,
and engineering sciences, 364(1845):2231–65.
Takagi, H., Mas, E., and Koshimura, S. (2014). Analysis
of the evacuation behavior in Natori, Yuriage during
the Great East Japan Earthquake Tsunami. In An-
nual Meeting of the Tohoku Branch Technology Re-
search Conference, Japan Society of Civil Engineers,
volume 3, pages 2011–2012.
Wijerathne, M., Melgar, L., Hori, M., Ichimura, T., and
Tanaka, S. (2013). HPC Enhanced Large Urban
Area Evacuation Simulations with Vision based Au-
tonomously Navigating Multi Agents. Procedia Com-
puter Science, 18:1515–1524.
Wilensky, U. (2001). Modeling Nature’s Emergent Patterns
with Multi-agent Languages. In EuroLogo, pages 1–9,
Linz, Austria.
SIMULTECH2014-4thInternationalConferenceonSimulationandModelingMethodologies,Technologiesand
Applications
254