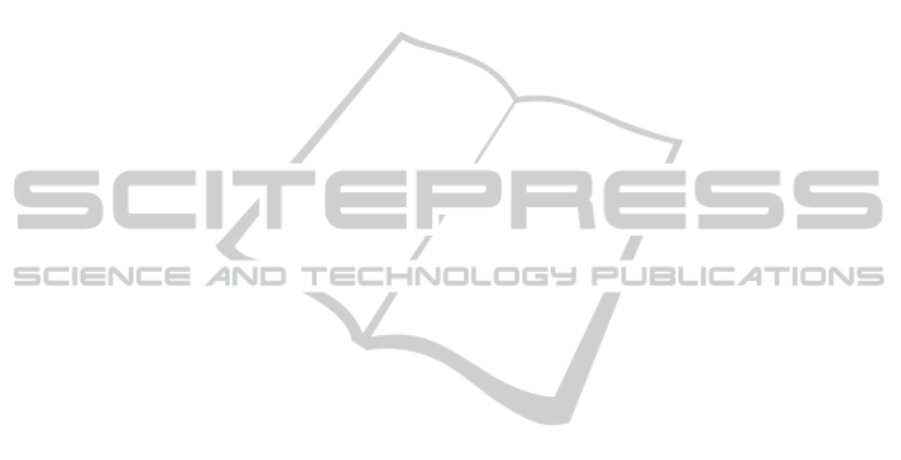
REFERENCES
Ae, M., Tang, H., and Yokoi, T. (1992). Estimation of in-
ertia properties of the body segments in japanese ath-
letes. Society of Biomechanisms Japan, 11:23–33. (in
Japanese).
Ben-Sira, D., Ayalon, A., and Tavi, M. (1995). The effect
of different types of strength training on concentric
strength in women. Journal of Strength and Condi-
tioning Research, 9(3):143–148.
Blazevich, A., Cannavan, D., Coleman, D., and Horne,
S. (2007). Influence of concentric and eccentric re-
sistance training on architectural adaptation in hu-
man quadriceps muscles. Journal of Applied Physi-
ology, 103(5):1565–1575. DOI: 10.1152/japplphys-
iol.00578.
Cadore, E., Gonzalez-Izal, M., Pallares, J., Rodriguez-
Falces, J., Hakkinen, K., Kraemer, W., Pinto, R., and
Izquierdo, M. (2014). Muscle conduction velocity,
strength, neural activity, and morphological changes
after eccentric and concentric training. Scandina-
vian Journal of Medicine and Science In Sports. doi:
10.1111/sms.12186.
Gonzalez-Izal, M., Cadore, E., and Izquierdo, M. (2014).
Muscle condition velocity, surface electromyography
variables, and echo intensity during concentric and ec-
centric fatigue. Muscle and Nerve, 49(3):389–397.
Hahn, D., Seiberl, W., Schmidt, S., Schweizer, K., and
Schwirtz, A. (2010). Evidence of residual force en-
hancement for multi-joint leg extension. Journal of
Biomechanics, 43(8):1503–1508.
Kellis, E., Arambatzi, F., and Papadopoulos, C. (2005). Ef-
fects of load on ground reaction force and lower limb
kinematics during concentric squats. Journal of Sports
Sciences, 23(10):1045–1055.
Kikuchi, T., Oda, K., Ohyama, Y., Isozumi, S., and Furusho,
J. (2009). Development of isokinetic exercise system
using high performance mr fluid brake. IEEE Interna-
tional Conference on Mechatronics.
Moromugi, S., Yoon, S., Kim, S., Tanaka, M., Ohgiya, Y.,
Matsuzaka, N., and Ishimatsu, T. (2006). A training
machine with dynamic load-control function based
on muscle activity information. Artificial Life and
Robotics, 10(2):126–130.
Nikitczuk, J., Weinberg, B., Canavan, P., and Mavroidis, C.
(2010). Active knee rehabilitation orthotic device with
variable damping characteristics implemented via an
electrorheological fluid. IEEE/ASME Transactions on
Mechatronics, 15(6):952–960.
Robertson, D., Wilsom, J., and Pierre, T. (2008). Lower
extremity muscle functions during full squats. Journal
of Applied Biomechanics, 24(4):333–339.
Seger, J., Arvidsson, B., and Thorstensson, A. (1998). Spe-
cific effects of eccentric and concentric trainig on
muscle strength and morphology in humans. Euro-
pean Journal of Applied Physiology, 79:49–57.
Shufang, D., Lu, K., Sun, J., and Rudolph, K. (2006). Adap-
tive force regulation of muscle strengthening rehabil-
itation device with magnetorheological fluids. IEEE
Transactions on Neural Systems and Rehabilitation
Engineering, 14(1):55–63.
icSPORTS2014-InternationalCongressonSportSciencesResearchandTechnologySupport
176