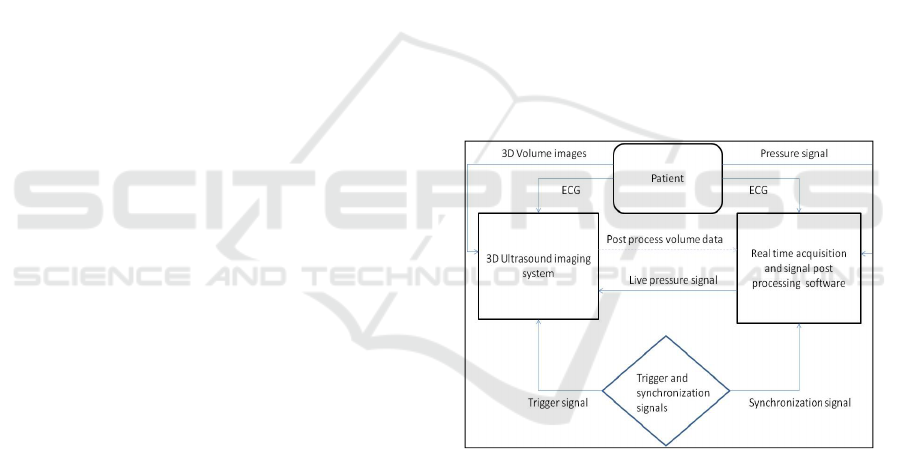
3D Echo and Invasive Pressure Synchronization
Generating Real Time, Multi Cycle Pressure Volume Loops
Dariusz Mroczek
1
, Kyong-Jin Lee
1
Juan Pablo Sandoval
1
, Helene Houle
2
,
Andreea Dragulescu, Lee Benson
1
and Rajiv R. Chaturvedi
1
1
Hospital for Sick Children, Toronto, Canada,
2
Siemens Medical Solutions USA, Inc., Ultrasound Division, Malvern, U.S.A.
1 BACKGROUND
The ventricular pressure-volume loop (PVL) relates
intracardiac pressure changes as a function of
volume changes during the cardiac cycle and is a
convenient method to understand major
determinants of myocardial performance. Acquiring
PVL is not simple in human models, the main
limitation being accurate volume measurement.
Traditionally PVL is obtained using conductance
catheterization which is based on the measurement
of the electrical conductivity of the blood volume by
placing a multiple electrode catheter along the long
axis of the ventricle (either right or left) during
catheterization and delivering an alternating current
between the most proximal and distal electrodes. -1-
Although conductance catheterization is considered
the gold standard for pressure volume relationship
acquisitions, this technology and equipment is
difficult to use and time consuming.
There has been considerable development in
three-dimensional echocardiography and more
recently display of cardiac structures in real time
rather than as offline reconstructed images from
multiple 2D echocardiographic slices. An important
clinical application of three-dimensional real-time
echocardiography (3D-RTE) includes delineation of
ventricular morphology and volume quantification -
2,3-
2 OBJECTIVES
Our objective was to explore the feasibility of using
3D-RTE together with cardiac catheterization to
determine ventricular function derived from PVL.
We established that success of our experiment
depends on several components and overall
reproducibility of our technique. Consequently we
identified signal synchronization (volume and
pressure), optimal sampling rate and the possibility
of multicycle acquisition as crucial requirements.
3 METHODS
3.1 Data Flow Diagram
3.2 Equipment
During cardiac catheterization ventricular volume
was attained by 3D RTE using the Siemens Acuson
SC2000 ultrasound system (Siemens Medical
Solutions USA Inc., Mountain View, CA) with a
4Z1c real-time volume imaging transducer (2.8
MHz). The SC2000 has a unique ability to produce
up to about 40 complete volumes per second in a
true real time acquisition mode. It is capable of
forming 64 parallel beams in real time and
processing of 160M voxels per second. Volume data
sets are free of multi-cycle averaging, regional
interpolation and “stitching” interference.
Ventricular pressure was obtained by a high
fidelity pressure cathether (Micro-Tip®, Millar,
Houston, Texas) which was advanced into the left or
right ventricle. Millar catheter frequency response is
greater than 10 kHz and the pressure signal was
acquired at 16 bit resolution by DT9804 (Data
Translation Inc) analog to digital converter.
All pressure, trigger and ECG signals were
recorded with Notocord hemodynamic software
(Notocord Systems, France, v 4.2).
Mroczek D., Lee K., Pablo Sandoval J., Houle H., Dragulescu A., Benson L. and R. Chaturvedi R..
3D Echo and Invasive Pressure Synchronization - Generating Real Time, Multi Cycle Pressure Volume Loops.
Copyright
c
2014 SCITEPRESS (Science and Technology Publications, Lda.)