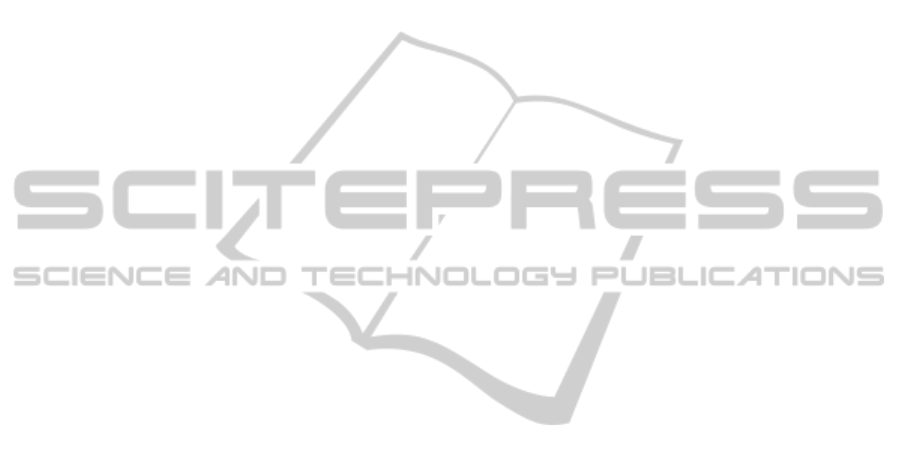
Universal Access in Human-Computer Interaction.
User and Context Diversity (pp. 253-258). Berlin:
Springer.
Bersak, D., McDarby, G., Augenblick, N., McDarby, P.,
McDonnell, D., McDonald, B. and Karkun, R. (2001).
Intelligent Biofeedback Using an Immersive
Competitive Environment. In Abowd, G. D., Brumitt,
B. and Shafer, S. (Eds.), UbiComp 2001-Lecture Note
in Computer Science (Vol. 2201). Heidelberg,
Germany: Springer.
Bremner, F. D. (2012). Pupillometric Evaluation of the
Dynamics of the Pupillary Response to a Brief Light
Stimulus in Healthy Subjects. Investigative
Ophthalmology & Visual Science, 53, 7343-7347.
doi: 10.1167/iovs.12-10881.
Crider, A. and Lunn, R. (1971). Electrodermal Lability as a
Personality Dimension, Journal of Experimental
Research in Personality, 5, 145-150.
Ehlers, J., Georgi, J. and Huckauf, A. (in press). Improving
Voluntary Pupil Size Changes for HCI.
Ekman, I., Poikola, A., Mäkäräinen, M., Takala, T. and
Hämäläinen, P. (2008). Voluntary Pupil Size Change as
Control in Eyes Only Interaction. Proceedings of the
2008
th
Symposium on Eye Tracking Research &
Applications, 115-118.
Fredrickson, B. L., Mancuso, R. A., Branigan, C., and
Tugade, M. M. (2000). The Undoing Effect of Positive
Emotions. Motivation and Emotion, 24(4), 237-258.
Hess, E. H. (1972). Pupillometrics. In Greenfield, N.S. and
Sternbach, R.A. (Eds.), Handbook of Psychophysiology
(pp. 491-531). New York, NY: Holt, Rinehart &
Winston.
Hyönä, J., Tommola, J. and Alaja, A. M. (1995). Pupil
Dilation as a Measure of Processing Load in
Simulataneous Interpretation and Other Language
Tasks. The Quarterly Journal of Experimental
Psychology, 48(A), 598-612.
Jacob, R. J. K. (1996). The Future of Input Devices. ACM
Computing Surveys, 28, 177-179.
Janisse, M. P. (1974). Pupil Size, Affect and Exposure
Frequency. Social Behavior and Personality, 2, 125-
146.
Laeng, B. and Sulutvedt, U. (2013). The Eye Pupil Adjusts
to Imaginary Light, Psychological Science, 27.
doi: 10.1177/0956797613503556.
Larsen, J. T., Berntson, G. G., Poehlmann, K. M., Ito, T. A.
and Cacioppo, J. T. (2000). The Psychophysiology of
Emotion. In Lewis, M. and Haviland-Jones, J. M.
(Eds.), Handbook of Emotions (2
nd
ed.) (pp.173-191).
New York, NY: Guilford Press.
Loewenfeld, I. E. (1966). Comment on Hess’ Findings.
Survey of Opthalmology, 11, 291-294.
Loewenfeld, I. E. (1993). Anatomy and Physiology. In
Loewenfeld, I. E. and Lowenstein, O. (Eds.), The Pupil:
Anatomy, Physiology, and Clinical Applications (pp.
498-510). Iowa City: Iowa State University Press.
Lowenstein, O., Feinberg, R. and Loewenfeld, I. E. (1963).
Pupillary Movements During Acute and Chronic
Fatigue Investigative Ophthalmology & Visual Science,
2(2), 138-157.
Meichenbaum, D. (1976). Cognitive Factors in
Biofeedback Therapy. Biofeedback and Self-
Regulation, 1(2), 201-216.
Naveteur, J., Buisine, S. and Gruzelier, J. H. (2005). The
Influence of Anxiety on Electrodermal Responses to
Distractors. International Journal of Psychophysiology,
56(3), 261-269.
Norris, C. J., Larsen, J. T. and Cacioppo, J. T. (2007).
Neuroticism Is Associated with Larger and More
Prolonged Electrodermal Responses to Emotionally
Evocative Pictures. Psychophysiology, 44(5), 823-826.
Palinko, O., Kun, A.L., Shyrokov, A. and Heeman, P.
(2010). Proceedings of the 2010
th
Symposium on Eye-
Tracking Research & Applications, 141-144.
Partala, T. and Surakka, V. (2003). Pupil Size Variation as
an Indication of Affective Processing. International
Journal of Human-Computer Studies, 59, 185-198.
doi: 10.1016/S1071-5819(03)00017-X.
Peavler, W.S. (1974). Pupil Size, Information Overload,
and Performance Differences. Psychophysiology,
11(5), 559-566.
doi: 10.1111/j.1469-8986.1974.tb01114.x.
Sakakibara, M., Takeuchi, S. and Hayano, J. (1994). Effect
of Relaxation Training on Cardiac Parasympathetic
Tone. Psychophysiology, 31(3), 223-228.
Simpson, H. M. and Paivio, A. (1968). Effects on Pupil Size
of Manual and Verbal Indicators of Cognitive Task
Fulfilment. Attention, Perception, & Psychophysics,
3(3), 185-190.
Winn, B., Whitaker, D., Elliott, D. B. and Phillips, N. J.
(1994). Factors Affecting Light-Adapted Pupil Size in
Normal Human Subjects. Investigative Ophthalmology
& Visual Science, 35(3), 1132-1137.
Yu, M., Kautz, M. A., Thomas, M. L., Johnson, D.,
Hotchkiss, E. R. and Russo, M. B. (2007). Operational
Implications of Varying Ambient Light Levels and
Time-Of-Day Effects on Saccadic Velocity and
Pupillary Light Reflex. Ophthalmic and Physiological
Optics, 27(2), 130-141.
doi: 10.1111/j.1475-1313.2006.00450.x.
PhyCS2015-2ndInternationalConferenceonPhysiologicalComputingSystems
12