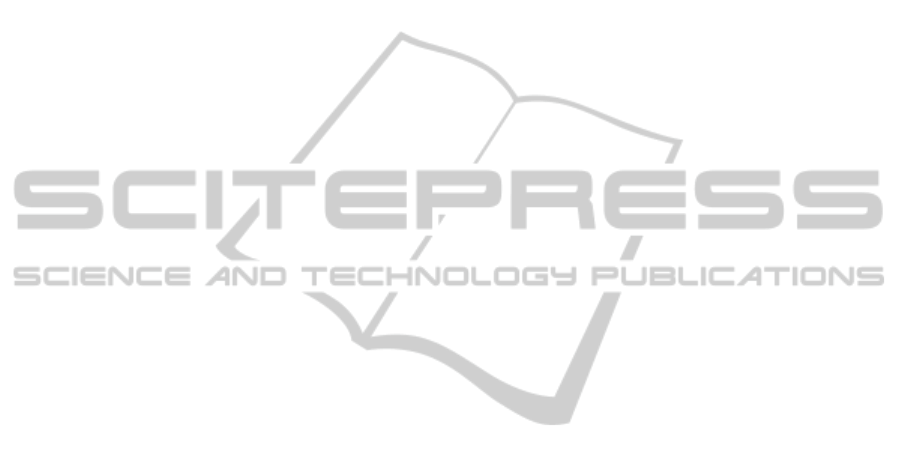
parameters and also sample inhomogeneity and
matrix effects may lead to nonlinearity in the
calibration curves, affecting the analytical precision
and consequently lowering the accuracy of
measurement by LIBS technique.
In general, sample inhomogeneity can be
categorized into two classes: bulk non-uniformity
and non-representative surface composition. The
first can be dealt with by averaging results of
multiple measurements taken over different parts of
the sample. Samples whose outer layers don’t
represent bulk composition can be probed by making
crater with depth ranging from few µm to 10-20 µm
depending on the laser parameters. This will usually
solve the problem of non representative surface
composition. Low ED-XRF, as mentioned before,
can’t probe such depth.
Matrix effect corresponds to the change in the
signal intensity of a specific element with variation
in the concentration of one or more elements
forming the matrix even though the overall
elemental concentration remains constant in the
sample. One approach to correct such effects is to
use internal standard principle i.e. to consider the
ratio of the intensity of analytical spectral line to that
of another component of the sample which has fixed
or known concentration because even if the
measured values change significantly, their ratio
alter a little (Haider et al. 2014). As LIBS offers
immense possibilities for spectroscopic analysis,
significant efforts are given to develop new
calibration methods (for a review, see (Zorov et al.
2010)) to correct LIBS measurements and increase
its ability of quantitative analyses.
Although LIBS is a standalone analytical
method, over the years, it has been combined with
other analytical methods to enhance performance for
selected applications. One such example is LIBS-
LIF (Laser Induced Fluorescence) where free atoms
in the plasma formed by LIBS laser are excited with
second laser beam tuned at specific frequency to
induce some transition in atoms and this method,
though not have multi-elemental detection
capability, has higher sensitivity to detect single
specific element in the sample. This has been
reflected in spectro-chemical analysis of metals in
soil (Hilbk-Kortenbruck et al., 2001).
In this paper, we report a method for determining
the carat values of gold in general, and in particular
of gold ornaments, by combining LIBS with low
energy dispersive XRF. This technique was also
adopted by Pouzar et al., 2011, for quantitative LIBS
analysis of vanadium in samples of hexagonal
mesoporous silica catalysts. One can thus bypass
the limitations, which both the systems suffer
individually, and enhance the detection ability of
impurity content in gold ornaments using calibration
curves obtained by correlating LIBS and XRF data.
It has been shown that improved accuracy of
determination of caratage of gold ornaments of any
size and shape are possible by using these
calibration curves and comparatively more reliable
results can be obtained from the LIBS technique.
2 EXPERIMENTAL
Suisse gold bar (24 carat) obtained from Singapore
Bullion market and gold ornaments: 3 finger rings
with known carat values, as determined by ED-XRF
technique, of 22.024K, 20.912K and 18.507K, a
round solid earring (20.495K), a round flat pendant
(18.807K), a curved ear-top (18.649K) and a stone
studded ring (8.040K) were used as samples. The
thickness of the targets was different for different
samples depending upon the type of ornaments.
Later an ornament of unknown carat value was used
for verification of the proposed method. It was
evident from XRF analysis that goldsmiths mostly
use copper and silver, as impurity to gold alloys for
obtaining desired color, shine and solidity of the
ornaments. The percentage content of impurity
elements (Cu and Ag) in the samples were
ascertained by low energy dispersive XRF. Other
elements which were present in trace amount were
Ni, Cd and Zn. That’s why in our work we have
mainly focused on establishing two separate
calibration curves; one for copper and another for
silver as the inclusion of other base metals were
negligible. These calibration curves were used to
estimate the impurity content of the gold ornament
of unknown caratage to verify the applicability of
this technique. The concentrations of constituent
elements in the gold samples were obtained by
desktop energy dispersive XRF spectrometer (EDX
3600B) which is equipped with Tungsten anode X-
ray tube operated at 40 KeV and 80 µA and electro-
cooling UHRD detector which leads to good energy
linearity, energy resolution, spectral property and
high peak-background ratio
(http://www.skyrayxrf.com/ edx3600b). The LIBS
spectrometer used in the present work is shown
figure 1 (Haider et al., 2014). It has a pulsed Q-
switched Nd:YAG laser operating at 1064 nm with
pulse duration of 8ns and repetition rate of 10 Hz.
But we have used harmonic generator that has a
nonlinear Potassium Dihydrophosphate (KDP)
crystal to obtain the 2
nd
harmonic at 532 nm with
PHOTOPTICS2015-InternationalConferenceonPhotonics,OpticsandLaserTechnology
42