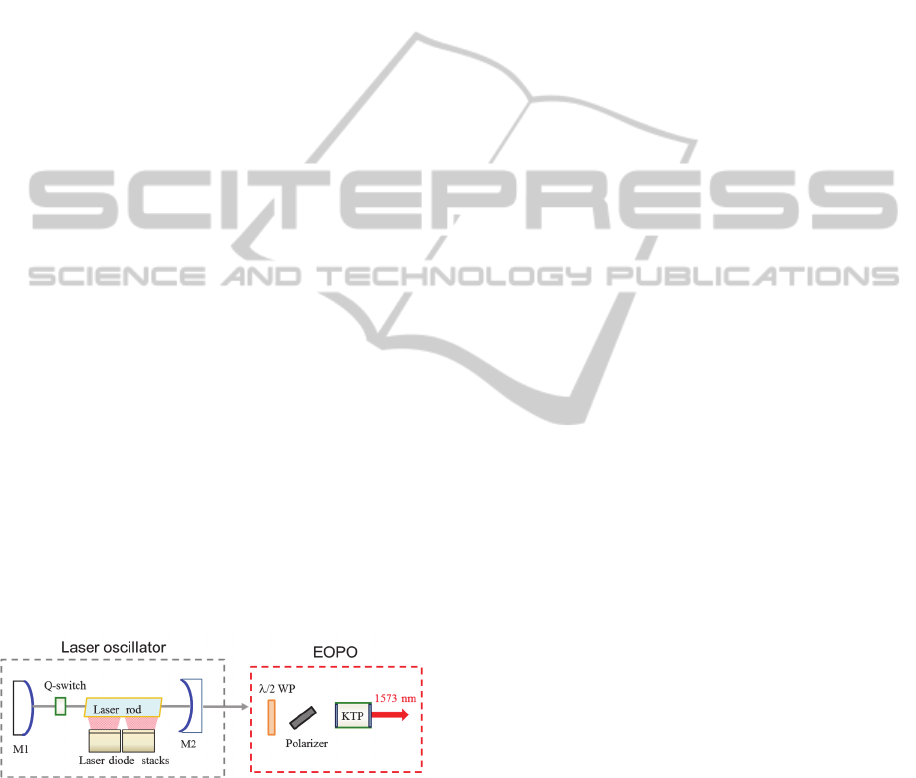
mirrors, respectively. The M
1
mirror was coated for
high reflection at 1064 nm on the convex surface.
The M
2
mirror was coated for partial reflection at
1064 nm on the concave surface. The gain medium
was a 1.0 at. % Nd:YAG crystal with a length of 25
mm, and cut with 2°-wedged end facets to avoid
etalon effects. Both end facets of the laser crystal
were coated for anti-reflection at 1064 nm, and the
pump face was coated for anti-reflection at 808 nm.
The diode stacks were placed close to the lateral
surface of the laser crystal to have good pump
efficiency, and were driven to emit optical pulses of
300 µs to match the upper-level lifetime of Nd:YAG
laser crystal. The Cr
4+
:YAG crystal with the initial
transmission of 30%. The nonlinear crystal KTP
with a length of 25 mm was cut along x axis (θ = 90°
and φ = 0°) for type-II non-critically phase-matched
OPO. The pump face of the KTP crystal, acting as
the front mirror of the OPO cavity, was coated for
high transmission at 1064 nm and high reflection at
1573 nm. The other face of the KTP crystal was
coated for high reflection at 1064 nm and partially
reflection at 1573 nm, which enables a double pass
of the 1064-nm input light within the KTP crystal to
lower the threshold and to enhance the efficiency for
OPO conversion. Note that a slight misalignment of
the OPO cavity, replacing the need of an optical
isolator, was applied to prevent the feedback of
1064-nm input light into the laser oscillator. An anti-
reflection coated half-waveplate at 1064 nm and a
polarizer were combined to be a variable attenuator
for adjusting the input pulse energy at 1064 nm. All
crystals were wrapped with indium foil and mounted
in conductively cooled copper blocks. The pulse
temporal behavior was recorded by a LeCroy digital
oscilloscope (Wavepro 7100, 10 G samples/s, 1 GHz
bandwidth) with a fast InGaAs photodiode.
Figure 1: Experimental configuration for an extracavity
OPO with a monolithic KTP crystal pumped by the
passively Q-switched Nd:YAG/Cr
4+
:YAG laser oscillator
with a convex-concave resonator.
3 EXPERIMENTAL RESULTS
First, the QCW free-running operation without the
Cr
4+
:YAG crystal was performed to confirm the
reliability of the laser configuration. Figure 2 depicts
the experimental results of the output energy as a
function of the diode pump energy in the free-
running operation. The threshold pump energy is
approximately 64 mJ. With a diode pump energy of
298 mJ, the output energy at 1064 nm is 122 mJ,
corresponding to an optical-to-optical conversion
efficiency of 41%. The temporal shape of the laser
pulse, as showed in Fig. 3, reveals a train of spikes
caused by the relaxation oscillation. The overall
slope efficiency is found to be 54%. We then
inserted the Cr
4+
:YAG crystal into the laser
resonator to implement the passively Q-switched
Nd:YAG/Cr
4+
:YAG laser oscillator. The threshold
pump energy for the Q-switched operation is
measured to be about 227 mJ, and the output pulse
energy at 1064 nm is about 30 mJ, corresponding to
an optical-to-optical conversion efficiency of 13.2%.
Compared with the results of the passively Q-
switched Nd:YAG/Cr
4+
:YAG laser with a QCW
diode side-pumping system to date (Zendzian,
Jabczynski and Kwiatkowski, 2008; Afzal et al.,
1997; Sauder, Minassian and Damzen, 2006), the
energy extraction efficiency is the highest to our
knowledge thanks to the superior cavity design of a
convex-concave resonator.
A typical temporal shape of the laser pulse, as
depicted in Fig. 4, displays the mode-locked
modulation resulted from the multi-longitudinal
mode beating. The separation of the mode-locked
pulses is verified to be correspondent with the cavity
round-trip frequency of 1.36 GHz. The Q-switched
pulse envelope is approximately 6 ns. The Q-
switched mode-locked pulses enhance the peak
power, which is estimated to be up to 6.3 MW. The
laser beam quality was measured employing the z-
scan method. The beam width was evaluated by the
scanning knife-edge method. Figure 5 shows the
experimental results of the beam widths in the
horizontal and vertical directions as a function of the
position along the propagation direction,
respectively. The beam quality factors M
2
are
estimated to be 5.0 × 3.0 (horizontal × vertical).
We then employ the developed Nd:YAG laser
oscillator to explore the performance of the
extracavity OPO. The dependence of the output
pulse energy at 1.57 m on the input pulse energy at
1.06 m is shown in Fig. 6. The OPO threshold
energy is approximately 6.0 mJ. With maximum
available input energy of 30 mJ at 1.06 m, the OPO
output energy of 13.3 mJ is obtained, leading to a
high slope efficiency of 54.8%. The OPO conversion
efficiency is also presented in Fig. 7 as a function of
the input pulse energy at 1.06 m. With increasing
OverTen-millijouleEye-safeLaserGenerationbyExtra-cavityOpticalParametricOscillatorDrivenwithaDiode-pumped
Nd:YAG/Cr4+:YAGQ-SwitchedLaser
173