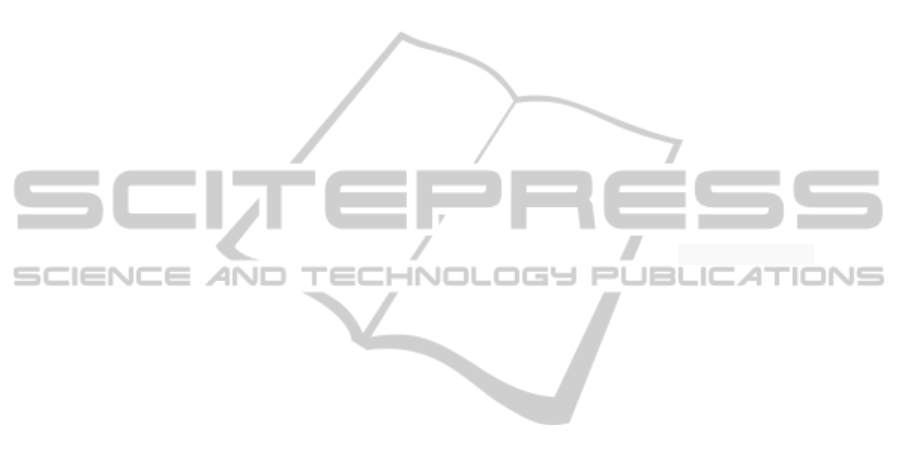
Oscarson, S., De Greve, H., Knight, S.D., 2006. The
affintiy of the FimH fimbrial adhesin is receptor-
driven and quasi-independent of Escherichia coli
pathotypes. Mol. Microbiol. 61(6), 1556-1568.
Bouguelia, S., Roupioz, Y., Slimani, S., Mondani, L.,
Casabona, M.G., Durmort, C., Vernet, T., Calmeczuk,
R., Livache, T., 2013. On-chip microbial culture for
the specific detection of very low levels of bacteria.
Lab Chip. 13, 4021-4032.
Campuzano, S., Orozco, J., Kagan, D., Guix, M., Gao, W.,
Sattayasamitsathit, S., Claussen, J.C., Merkoci, A.,
Wang, J., 2012. Bacterial isolation by lectin-modified
microengines. Nano Lett. 12, 396-401.
Chang, C-F., Pan, J-F., Lin, C-N., Wu, I-L., Wong, C-H.,
Lin, C-H., 2011. Rapid characterization of sugar-
binding specificty by in-solution proximity binding
with photosensitizers. Glycobiology 21(7), 895-902.
Chessa, D., Winter, M.G., Jakomin, M., Bäumler, A.J.,
2009. Salmonella enterica serotype Typhimurium Std
fimbriae bind terminal α (1,2)fucose residues in the
cecal mucosa. Mol. Microbiol. 71(4), 864-875.
Chu, Y.W., Engebretson, D.A., Carey, J.R., 2013.
Bioconjugated magnetic nanoparticles for the
detection of bacteria. J. Biomed. Nanotechnol. 9,
1951-1961.
Cowart, R.E., Lashmet, J., McIntosh, M.E., Adams, T.J.,
1990, Adherence of a virulent strain of Listeria
monocytogenes to the surface of a hepatocarcinoma
cell line via lectin-substrate interaction. Arch.
Microbiol. 153, 282-286.
Delehanty, J.B., Ligler, F.S., 2002. A microarray
immunoassay for simultaneous detection of proteins
and bacteria. Anal. Chem. 74, 5681-5687.
Fort, S., Birikaki, L., Dubois, M-P., Antroine, T., Samain,
E., Driguez, H., 2005. Synthesis of conjugatable
saccharide moieties of GM2 and GM3 glycosides by
engineered E. coli. Chem. Commun. 20, 2558-2560.
Gao, J., Liu, C., Wang, Z., Dong, S., 2010. Antibody
microarray-based strategies for detection of bacteria
by lectin-conjugated gold nanoparticle probes. Talanta
81, 1816-1820.
Gilmartin, N., O’Kennedy, R., 2012. Nanobiotechnologies
for the detection and reduction of pathogens. Enzym.
Microb. Techol. 50, 87-95.
Gu, H., Xu, K., Xu, C., Xu, B., 2006. Biofunctional
magnetic nanoparticles for protein separation and
pathogen detection. Chem. Commun. 941-949.
Lin, Y-Y.A., Chalker, J.M., Davis, B.G., 2010. Olefin
Cross-Metathesis on Proteins: Investigation of Allylic
Chalcogen Effects and Guiding Principles in
Metathesis Partner Selection. J. Am. Chem. Soc.
132(47), 16805-16811.
Mader, A., Gruber, K., Castelli, R., Hermann, B.A.,
Seeberger, P.H., Rädler, J.P., Leisner, M., 2012.
Discrimination of Escherichia coli strains using
glycan cantilever array sensors. Nanolett. 12, 420-423.
Mercey, E., Sadir, R., Maillart, E., Roget, A., Baleux, F.,
Lortat-Jacob, H., Livache, T., 2008. Polypyrrole
oligosaccharide array and surface plasmon resonance
imaging for the measurement of glycosaminoglycan
binding interactions. Anal. Chem. 80, 3476-3482.
Mondani, L., Roupioz, Y., Delannoy, S., Fach, P.,
Livache, T., 2014. Simultaneous enrichment and
optical detection of low levels of stressed Escherichia
coli O157:H7 in food matrices, J. Appl. Microbiol.
117(2), 537-546.
Nishida, Y., Mizuno, A., Kato, H., Yashiro, A., Ohtake,
T., Kobayashi, K., 2004. Stereo- and Biochemical
Profiles of the 5-6- and 6-6-Junction Isomers of α-D-
Mannopyranosyl [60]Fullerenes. Chem. Biodivers. 1,
1452-1464.
Roy, R., Laferriere, C.A., 1990. Synthesis of protein
conjugates and analogues of N-acetylneuraminic acid.
Can. J. Chem. 68, 2045-2054.
Safina, G., 2012. Application of surface plasmon
resonance for the detection of carbohydrates,
glyconjugates, and measurement of the carbohydrate-
specific interactions: a comparison with conventional
analytical techniques. Anal. Chim. Acta. 712, 9-29.
Scallan, E., Hoekstra, R.M., Angulo, F.J., Tauxe, R.V.,
Widdowson, M-A., Roy, S.L., Jones, J.L., Griffin,
P.M., 2011. Foodborne illness acquired in the United
States – Major pathogens. Emerg. Infect. Dis. 17(1), 7-
15.
Takeo, T., Imai, T., 1987. Synthesis of trisaccharide
glycosides related to nigeran. Carbohyd. Res. 165(1),
123-128.
Tallury, P., Malhotra, A., Byrne, L.M., Santra, S., 2010.
Nanobioimaging and sensing of infectious diseases.
Adv. Drug Deliv. Rev. 62, 424-437.
Vauzeilles, B., Dausse, B., Palmier, S., Beau, J-M., 2001.
A one-step β-selective glycosylation of N-acetyl
glucosamine and recombinant
chitooligosaccharides. Tetrahedron Lett. 42, 7567-
7570.
Velusamy, V., Arshak, K., Korostynska, O., Oliwa, K.,
Adley, C., 2010. An overview of foodborne pathogen
detection: in the perspective of biosensors. Biotechnol.
Adv. 28, 232-254.
Vermeer, H.J., van Dijk, C.M., Kamerling, J.P.,
Vliegenthart, J.F.G., 2001. Fucosylation of Linear
Alcohols: A Study of Parameters Influencing the
Stereochemistry of Glycosylation. Eur. J. Org. Chem.
1, 193-203.
BIODEVICES2015-InternationalConferenceonBiomedicalElectronicsandDevices
126