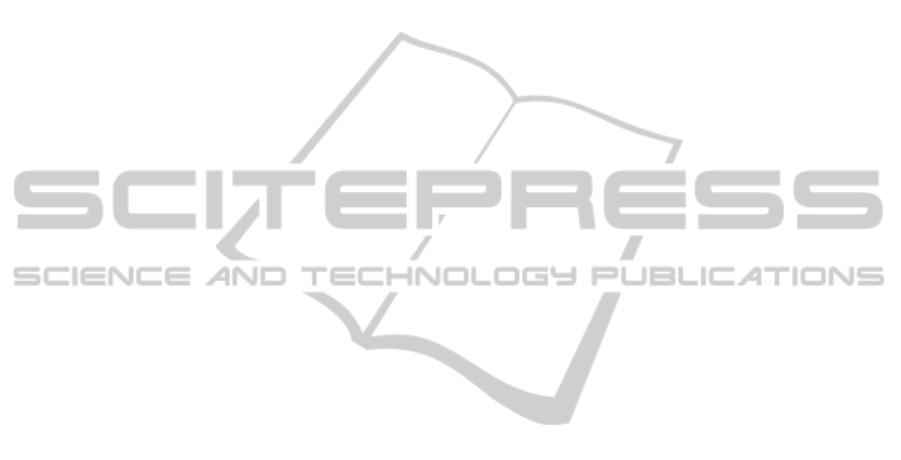
ACKNOWLEDGEMENTS
This work was supported by grants DEC-
2012/05/B/NZ2/01618 (JS, AN, PJ, PW, MKimmel)
and BKM/524/RAU1/2014/t.5 (MKardynska).
REFERENCES
Amman J. U., Haag C., Kasperczyk H., Debatin K. M.,
Fuld S. (2009) Sensitization of neuroblastoma cells for
TRAIL-induced apoptosis by NFkB inhibition, Int. J.
Cancer, 124, 1301–1311.
Ciocca D. R., Calderwood S. K. (2005) Heat shock
proteins in cancer: diagnostic, prognostic, predictive,
and treatment implications. Cell Stress Chaperones
10, 86–103.
Daugaard M., Rohde M, Jaattela M. (2007) The heat
shock protein 70 family: highly homologous proteins
with overlapping and distinct functions. FEBS Lett.
581, 3702–3710.
Domingo-Domènech J., Pippa R., Tápia M., Gascón P.,
Bachs O, Bosch M (2008) Inactivation of NF-κB by
proteasome inhibition contributes to increased
apoptosis induced by histone deacetylase inhibitors in
human breast cancer cells, Breast Cancer Research
and Treatment, 112(1), 53-62.
Fujimoto M., Nakai A. (2010) The heat shock factor
family and adaptation to proteotoxic stress, FEBS
Journal, 277, 4112–4125.
Janus P., Pakula-Cis M., Kalinowska-Herok M., Kashchak
N., Szołtysek K., Pigłowski W., Widlak W., Kimmel
M., Widlak P. (2011), NF-kB signaling pathway is
inhibied by heat shock independently of active
transcription factor HSF1 and increased levels of
inducible heat shock proteins, Genes to Cells, 16,
1168–1175.
Lee Titsworth W., Murad G. J., Hoh B. L., Rahman M
(2014) Fighting fire with fire: the revival of
thermotherapy for gliomas. Anticancer Res. 34(2),
565-574.
Lipniacki T., Paszek P., Brasier A. R., Luxon B., Kimmel
M (2004) Mathematical model of NF-kB regulatory
module. J Theor Biol, 228, 195-215.
Morimoto R. I. (2011) The heat shock response: systems
biology of proteotoxic stress in aging and disease.
Cold Spring Harb Symp Quant Biol. 76, 91-99.
Neznanov N., Komarov A. P., Neznanova L., Stanhope-
Baker P., Gudkov A. V. (2011) Proteotoxic stress
targeted therapy (PSTT): induction of protein
misfolding enhances the antitumor effect of the
proteasome inhibitor bortezomib. Oncotarget 2, 209–
221.
Peper A., Grimbergen C. A., Spaan J. A. E., Souren J. E.
M., van Wijk R. (1997) A mathematical model of the
hsp70 regulation in the cell. Int J Hyperth 14(1), 97–
124.
Petre I., Mizera A., Hyder C. L., Meinander A., Mikhailov
A., Morimoto R. I., Sistonen L., Eriksson J. E., Back
R. (2011) A simple mass-action model for the
eukaryotic heat shock response and its mathematical
validation. Nat. Comp. 10, 595–612.
Ran R., Lu A., Zhang L., Tang Y., Zhu H., Xu H., Feng
Y., Han C., Zhou G., Rigby A. C., Sharp F. R. (2004)
Hsp70 promotes TNF-mediated apoptosis by binding
IKK gamma and impairing NF-kappa B survival
signaling.
Genes Dev. 18, 1466–1481.
Rieger T. R., Morimoto R. I., HatzimanikatisV. (2005)
Mathematical Modeling of the Eukaryotic Heat-Shock
Response: Dynamics of the hsp70 Promoter,
Biophysical Journal, 88, 1646–1658.
Rybinski M., Szymanska Z., Lasota S., Gambin A. (2013)
Modelling the efficacy of hyperthermia treatment, J.
R. Soc. Interface, 10: 20130527.
Sheppard P. W., Sun X., Khammash M., Giffard R. G.
(2014) Overexpression of Heat Shock Protein 72
Attenuates NF-kB Activation Using a Combination of
Regulatory Mechanisms in Microglia. PLoS Comput
Biol 10(2): e1003471.
Sung M. H., Simon R. (2004) In silico simulation of
inhibitor drug effects on nuclear factor-kB pathway
dynamics. Mol. Pharmacol. 66, 70–75.
Szymanska Z., Zylicz M (2009) Mathematical modeling
of heat shock protein synthesis in response to
temperature change, J Theor Biol, 259, 562–569.
Wang Y., Paszek P., Horton C. A., Kell D. B., White M.
R. H., Broomhead DS, Muldoon MR (2011)
Interactions among oscillatory pathways in NF-kappa
B signaling, BMC Sys Biol, 5:23.
Wang Y., Paszek P., Horton C. A., Yue H., White M. R.
H., Kell D. B., Muldoon M. R., Broomhead D. S
(2012) A systematic survey of the response of a model
NF- signalling pathway to stimulation, J Theor Biol,
297, 137-147.
Westerheide S. D, Raynes R., Powell C., Xue B., Uversky
V. N. (2012) HSF transcription factor family, heat
shock response, and protein intrinsic disorder. Curr
Protein Pept Sci. 13(1), 86-103.
Yamamoto Y., Gaynor R. B. (2001) Therapeutic potential
of inhibition of the NF-κB pathway in the treatment of
inflammation and cancer, J Clin Invest. 107(2), 135–
142.
Yang P., Zhou T. (2013) Receptor-dependent sensitivity of
NF-κB to low physiological level, J Biol Sys, 21(3):
1350018.
Zambrano S., Bianchi M. E., Agresti A. (2014), A simple
model of dynamics reproduces experimental
observations, J Theor Biol, 347, 44-53.
Zanotto-Filho A., Braganhol E., Schröder R., de Souza L.
H. T., Dalmolin R. J. S., Bittencourt Pasquali M. A.,
Gelain D. P., Battastini A. M. O., Moreira J. C. F.
(2011) NFκB inhibitors induce cell death in
glioblastomas, Biochemical Pharmacology, 81(3),
412-424.
BIOINFORMATICS2015-InternationalConferenceonBioinformaticsModels,MethodsandAlgorithms
206