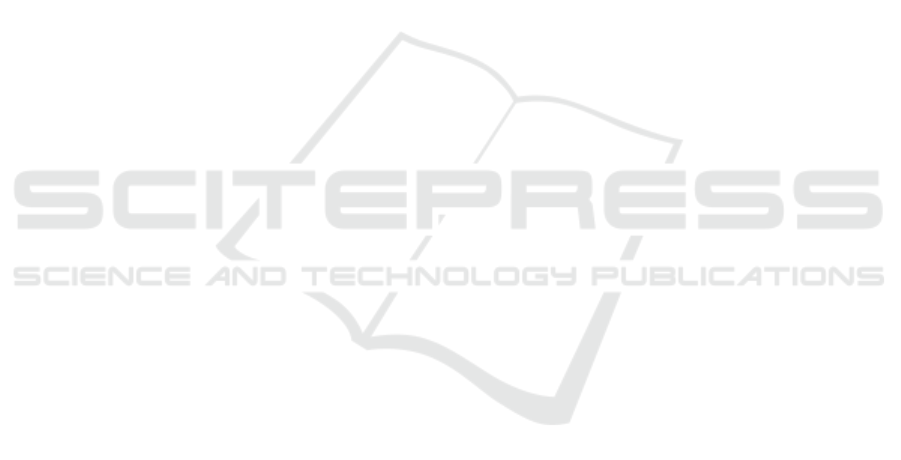
Micro Sensors for Real-time Monitoring of Mold Spores and Pollen
Kei Tsuruzoe and Kazuhiro Hara
Department of Electrical and Electronics Engineering, School of Engineering, Tokyo Denki University
5 Senjyu-Asahicho, Adachiku, Tokyo 120-8551, Japan
Keywords: Mold Spore, Pollen, Real–time Monitoring, Micro Sensor, Semiconductor Thin Film, Metal Oxide.
Abstract: Organic airborne particles such as mold spores and pollen cause a variety of diseases. Two types of micro
sensors for real-time monitoring of such organic airborne particles have been developed using
semiconductor thin-film. A basic type thin-film sensor has a simple configuration with a double-layered
sensing film deposited on an alumina substrate. A MEMS type sensor is composed of two parts: a sensing
element and a micro heater. Both parts are fabricated by using thin film technology, IC fabrication process
and micromachining technique. The double-layered sensing film is deposited on a diaphragm formed on a
Si substrate. A thin film heater is placed in parallel at a distance of about 50 μm. The resistance of both
sensors steeply decreases and then recovers to the initial value when a mold spore or a grain of pollen
adheres to the surface of the sensing film and burns on it. The resistance change and the recovery time
depend on the size of the organic airborne particles. Thus it is possible to identify the species of the particle
by the developed sensors. The sensors offer simple and inexpensive method to monitor organic airborne
materials.
1 INTRODUCTION
Many people fall in pneumonia by inhalation of
mold spores such as aspergillus fumigatus. At the
same time, the number of people who are allergic to
organic airborne particles such as pollen and house
dust has been increasing. Although human noses are
sensitive to some toxic gases and odors such as burnt
odor and bad smell, human noses cannot detect
organic airborne particles. Therefore, it is required to
develop sensors for these airborne particles.
Some methods have been developed to monitor
airborne
materials so far. Various types of samplers
are usually used to count the number of airborne
mold spores (Hoisington et al., 2014; Whyte et al.,
2007). However, they are disadvantageous in that it
takes several days to culture them on agar. The
gravitational method by a Durham’s sampler is a
common one to obtain the number of pollen
(Konishi et al., 2014). It also takes a lot of time to
get the number because they are observed by the
human eye through an optical microscope. These
two methods are not fit for real-time monitoring of
airborne materials. Particle counters with laser optics
are sometimes used (Weber et al., 2012). However,
they cannot distinguish organic airborne particles
from inorganic particles such as ashes and sands. In
addition, they are complex and expensive in general.
This paper describes novel, inexpensive micro
sensors that are capable of detecting organic
airborne particles such as mold spores and pollen.
Two types of micro sensors have been developed: a
basic type sensor and a MEMS type sensor. The
former has a simple structure with a relatively large
sensing area that is suitable for detection of larger
airborne particles. The latter has a smaller sensing
area that is better suited for detection of smaller
airborne particles.
Both sensors have configurations which are
similar to thin-film gas sensors (Brunet et al., 2012;
Sharma et al., 2011). In addition, similar sensing
materials based on semiconductor metal oxides such
as SnO
2
and Fe
2
O
3
were used for the developed
sensors. In general, these metal oxides are stable at
elevated temperatures and their resistance changes
by the redox reaction on the surface when a reducing
gas or an oxidizing gas is introduced. In ordinary gas
sensors, greater sensing area is better because it
increases the sensitivity to these gases. In this study,
however, the sensing area was reduced to fit for
detection of fine airborne particles.
174
Tsuruzoe K. and Hara K..
Micro Sensors for Real-time Monitoring of Mold Spores and Pollen.
DOI: 10.5220/0005279301740179
In Proceedings of the International Conference on Biomedical Electronics and Devices (BIODEVICES-2015), pages 174-179
ISBN: 978-989-758-071-0
Copyright
c
2015 SCITEPRESS (Science and Technology Publications, Lda.)