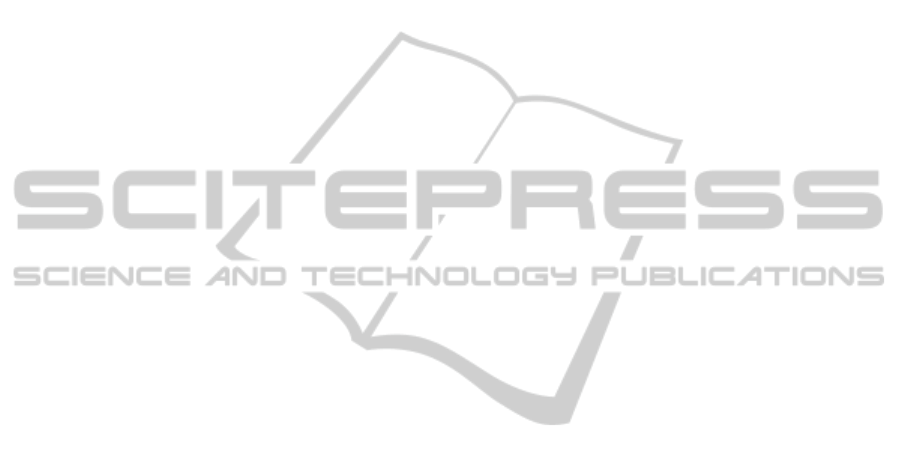
1) to the action of the TA muscle for adjusting joint
position after dorsiflexion trend held by TA.
4 DISCUSSION
Despite that the research's participant didn´t know
the exact time of the perturbation, he knew that the
disturbance would happen at any time. In this
situation, Stokes et al. (2000) suggest that pre-
activation occurs in the muscles of the participant
who is subjected to a disturbance of equilibrium,
which is also associated with an increased sensitivity
of the muscle spindle, causing changes in length and
increasing the probability of responding to a sudden
muscle disorder.
Cort et al. (2013) analyzed the contributions of
the muscles of the spine due to balance's disorders,
they concluded that the participants also performed
muscle contractions prior to perturbation. This
enhances the performance of the neuromuscular
system to responses to sudden balance's disorders,
increasing the sensitivity of the receptors responsible
for detecting motion (Cort et al., 2013).
Figure 2 shows that there is an increase in SO
muscle tone prior to perturbation. However, after the
disturbance, the EMG RMS envelop signal
undergoes a sudden attenuation compared to the pre-
perturbation. We believe that this reduction is
associated with transmission's inhibition of the
action potential to the muscle.
Crone et al. (1994), for example, conducted
physical training aimed at increasing the response of
reciprocal inhibition in healthy individuals and in
individuals with musculoskeletal disorders (Geertsen
et al., 2008).
This phenomenon can be explained by the
occurrence of the stretch reflex in the TA muscle
that has the effect of reciprocal inhibition of the
antagonist muscle group to its action.
In a study by Robinovitch and Murnaghan
(2013), using a linear motor connected to a mobile
platform to promote a controlled perturbation, 14
young women were evaluated in three conditions
(forward swing, back swing and static). The results
suggest that the amount of latency (defined as the
time between the onset of the disturbance and the
onset of muscle contraction) in all seven analyzed
muscles (Erector Right Column, Rectus Abdominis,
Soleus, Gastrocnemius, Tibialis Anterior, Rectus
Femoris and Biceps Femoris) seemed to occur
earlier when participants performed a static position.
The authors also concluded that the action of the use
muscles for recovery of the balance are adapted
depending on the nature of the disturbance and the
requested task.
Regarding aging, the work of Piirainen et al.
(2013) monitored EMG signal of muscles SO, TA
and Gastrocnemius of 9 young adults and 10 older
adults in the recovery of postural equilibrium after a
disturbance in the anterior-posterior and posterior-
anterior directions. The authors noted that, due to
aging a decrease in postural control occurs. The
action of SO and TA muscles may not be functional
for maintaining equilibrium in the face of
perturbations in different directions of evaluated
perturbations, indicating a distinct global motor
strategy to balance recovery, and yet, with activation
of antagonistic muscle groups for different
directions of perturbations.
5 CONCLUSIONS
It can be concluded that in the situation of balance
perturbation in posterior-anterior direction, the TA
muscle contracts before the SO, and the SO has pre-
activation, which is inhibited due to the stretch
reflex that occurs in the TA muscle.
REFERENCES
Cort, J. A., Dickey, J. P. & Potvin, J. R. 2013. Trunk
muscle contributions of to L4-5 joint rotational
stiffness following sudden trunk lateral bend
perturbations. J Electromyogr Kinesiol, 23, 1334-42.
De Medeiros, V. M. L., De Lima, F. M. R. & Di Pace, A.
M. 2010. Equilíbrio, controle postural e suas
alterações no idoso.
Duarte, M. & Freitas, S. 2010. Revisão sobre
posturografia baseada em plataforma de força para
avaliação do equilíbrio. Rev bras fisioter, 14, 183-92.
Geertsen, S. S., Lundbye-Jensen, J., Nielsen, J. B., 2008.
Increased central facilitation of antagonist reciprocal
inhibition at the onset of dorsiflexion following
explosive strength training. Journal of appl physiol;
105 (3): 915-22.
Horak, F. B. 2006. Postural orientation and equilibrium:
what do we need to know about neural control of
balance to prevent falls? Age Ageing, 35 Suppl 2, ii7-
ii11.
Horak, F. B., Shupert, C. L. & Mirka, A. 1989.
Components of postural dyscontrol in the elderly: a
review. Neurobiology of aging, 10, 727-738.
Linford, C. W., Hopkins, J. T., Schulthies, S. S., Freland,
B., Draper, D. O. & Hunter, I. 2006. Effects of
neuromuscular training on the reaction time and
electromechanical delay of the peroneus longus
BalancePerturbationLeadsaStretchingReflexiononTibialisAnteriorMuscle
327