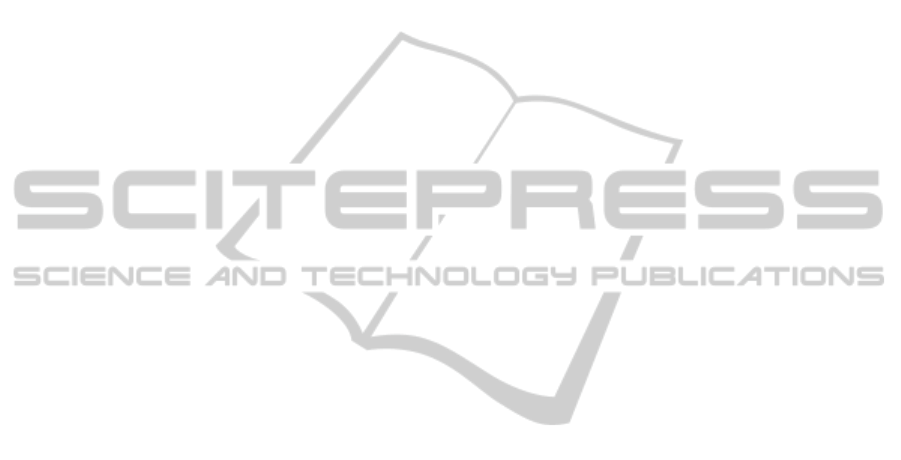
REFERENCES
Aldrich, T. K., Shander, A., Chaudhry, I., and Nagashima,
H. (1986). Fatigue of isolated rat diaphragm: role of
impaired neuromuscular transmission. Journal of Ap-
plied Physiology, 61(3):1077–1083.
Brooks, S. V. and Faulkner, J. A. (1988). Contractile prop-
erties of skeletal muscles from young, adult and aged
mice. Journal of Physiology, 404:71–82.
Del Prete, Z., Musaro`, A., and Rizzuto, E. (2008).
Measur- ing mechanical properties, including isotonic
fatigue, of fast and slow MLC/mIgf-1 transgenic
skeletal mus- cle. Annals of biomedical engineering.
Derave, W., Van Den Bosch, L., Lemmens, G., Eijnde, B.
O., Robberecht, W., and Hespel, P. (2003). Skele- tal
muscle properties in a transgenic mouse model for
amyotrophic lateral sclerosis: effects of creatine treat-
ment. Neurobiology of disease, 13(3):264–272.
Dupuis, L., Gonzalez de Aguilar, J. L., Echaniz-Laguna,
A., Eschbach, J., Rene, F., Oudart, H., Halter, B.,
Huze, C., Schaeffer, L., Bouillaud, F., and Loeffler, J.
P. (2009). Muscle mitochondrial uncoupling
dismantles neuromuscular junction and triggers distal
degenera- tion of motor neurons. PloS one,
4(4):e5390.
Fischer, L. R., Culver, D. G., Tennant, P., Davis, A. A.,
Wang, M., Castellano-Sanchez, A., Khan, J., Polak,
M. A., and Glass, J. D. (2003). Amyotrophic lateral
sclerosis is a distal axonopathy: evidence in mice and
man. Experimental Neurology, 185(2):232–240.
Gurney, M. E., Pu, H., Chiu, A. Y., Canto, M. C. D., Pol-
chow, C. Y., Alexander, D. D., Caliendo, J., Hentati,
A., Kwon, Y. W., and Deng, H. X. (1994). Mo- tor
neuron degeneration in mice that express a hu- man
Cu,Zn superoxide dismutase mutation. Science,
264(5166):1772–1775.
Hegedus, J., Putman, C. T., Tyreman, N., and Gordon, T.
(2008). Preferential motor unit loss in the SOD1 G93A
transgenic mouse model of amyotrophic lateral
sclerosis. The Journal of physiology, 586(14):3337–
3351.
Lee, Y. I., Mikesh, M., Smith, I., Rimer, M., and
Thompson, W. (2011). Muscles in a mouse model of
spinal mus- cular atrophy show profound defects in
neuromuscu- lar development even in the absence of
failure in neu- romuscular transmission or loss of
motor neurons. De- velopmental biology, 356(2):432–
444.
Ling, K. K. Y., Lin, M.-Y., Zingg, B., Feng, Z., and Ko,
C.-P. (2009). Synaptic defects in the spinal and neu-
romuscular circuitry in a mouse model of spinal mus-
cular atrophy. PloS one, 5(11):e15457–e15457.
Ngo, S. T., Baumann, F., Ridall, P. G., Pettitt, A. N.,
Henderson, R. D., Bellingham, M. C., and Mc-
Combe, P. A. (2012). The relationship between
Bayesian motor unit number estimation and histo-
logical measurements of motor neurons in wild-type
and SOD1(G93A) mice. Clinical Neurophysiology,
123(10):2080–2091.
Personius, K. E. and Sawyer, R. P. (2006). Variability and
failure of neurotransmission in the diaphragm of mdx
mice. Neuromuscular disorders : NMD, 16(3):168–
177.
Turner, B. J. and Talbot, K. (2008). Transgenics, toxicity
and therapeutics in rodent models of mutant SOD1-
mediated familial ALS. Progress in neurobiology,
85(1):94–134.
Van Lunteren, E., Moyer, M., and Kaminski, H. J. (2004).
Adverse effects of myasthenia gravis on rat phrenic di-
aphragm contractile performance. Journal of Applied
Physiology, 97(3):895–901.
DevelopmentofanAutomatedSystemforExVivoMeasuringtheNeuroMuscularJunctionFunctionality
41