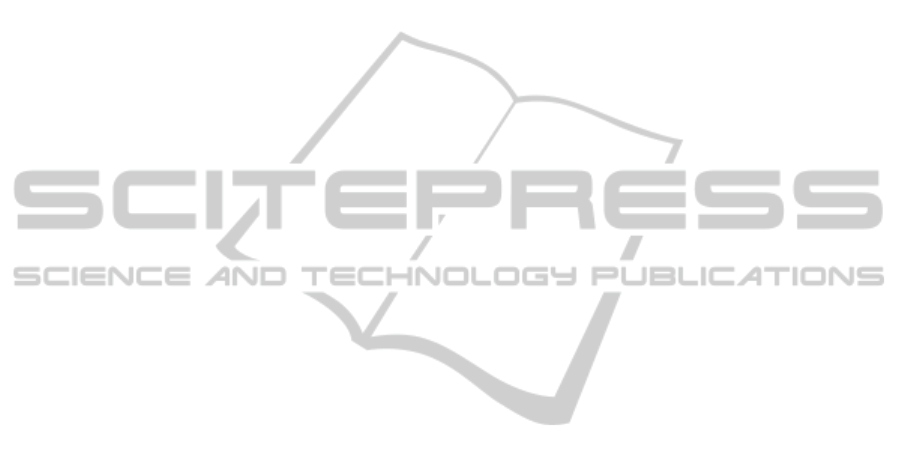
ACKNOWLEDGEMENTS
The authors would like to acknowledge support from
the China Scholarship Council (CSC). The authors
would also like to dedicate this work to the memory
of the recently deceased Professor Alfredo Martín
Mínguez.
REFERENCES
Abe, K., Lacroix, Y., Bonnell, L., Jakubczyk, Z., 1992.
Modal interference in a short fiber section: fiber length,
splice loss, cutoff, and wavelength dependences.
Journal of Lightwave Technology. vol. 10, no. 4, pp.
401-406.
Blahut, M., Kasprzak, D., 2004. Multimode interference
structures – properties and applications. Optica
Applicata. vol. XXXIV, no.4, pp. 573-587.
Chen, X., Horche, P.R., Minguez, A.M., 2014. DPSK
Signals Demodulation Based on a Multimode Fiber
with a Central Dip. In NOC 2014, Milan, Italy.
Chow, C.W., Tsang, H.K., 2005. Polarization-independent
DPSK demodulation using a birefringent fiber loop.
IEEE Photonics Technology Letters. vol. 17, no. 6, pp.
1313–1315.
Du, J., Dai, Y., Lei, G.K.P., Tong, W., Shu, C., 2010.
Photonic crystal fiber based Mach-Zehnder
interferometer for DPSK signal demodulation. Optics
Express. vol. 18, no. 8, pp. 7917-7922.
Gnauck, A.H., Winzer, P.J., 2005. Optical phase-shift-
keyed transmission. Journal of Lightwave Technology.
vol. 23, no. 1, pp. 115-130.
Hofmann, P., Mafi, A., Jollivet, C., Tiess, T.,
Peyghambarian, N., Schülzgen, A., 2012. Detailed
investigation of mode-field adapters utilizing
multimode-interference in graded index fibers. Journal
of Lightwave Technology. vol. 30, no. 14, pp. 2289-
2298.
Horche, P.R., López-Amo, M., Muriel, M.A., Martin-
Pereda, J.A., 1989. Spectral behavior of a low-cost all-
fiber component based on untapered multifiber unions.
IEEE Photonics Technology Letters. vol. 1, no. 7, pp.
184-187.
Horche, P.R., Muriel, M.A., Martin-pereda, J.A., 1989.
Measurement of transmitted power in untapered
multifibre unions: oscillatory spectral behaviour.
Electronics Letters. vol. 25, no. 13, pp. 843-844.
Kumar, A., Varshney, R.K., Antony, S., Sharma, P., 2003.
Transmission characteristics of SMS fiber optic sensor
structures. Optics Communications. vol. 219, no. 1-6,
pp. 215-219.
Lize, Y.K., Gomma, R., Kashyap, R., 2006. Low-cost
multimode fiber Mach-Zehnder interferometer for
differential phase demodulation. In SPIE 2006, San
Diego, USA.
Mohammed, W.S., Mehta, A., Johnson, E.G., 2004.
Wavelength tunable fiber lens based on multimode
interference. Journal of Lightwave Technology. vol. 22,
no. 2, pp. 469-477.
Pang, F., Liang, W., Xiang, W., Chen, N., Zeng, X.,
Chen, Z., and Wang, T., 2009, Temperature-
Insensitivity Bending Sensor Based on Cladding-
Mode Resonance of Special Optical Fiber, IEEE
Photonics Technology Letters, vol. 21, no. 2, pp. 76-
78.
Shao, M., Qiao, X., Fu, H., Li, H., Jia, Z., Zhou, H., 2014.
Refractive index sensing of SMS fiber structure based
Mach–Zehnder interferometer. IEEE Photonics
Technology Letters. vol. 26, no. 5, pp. 437-439.
Tripathi, S.M., Kumar, A., Marin, E., Meunier, J.-P., 2010.
Single-multi-single mode structure based band
pass/stop fiber optic filter with tunable bandwidth.
Journal of Lightwave Technology. vol. 26, no. 24, pp.
3535-3541.
Tripathi, S.M., Kumar, A., Varshney, R.K., Kumar, Y.B.P.,
Marin, E., Meunier, J.-P., 2009. Strain and
temperature sensing characteristics of single-mode-
multimode-single-mode structures. Journal of
Lightwave Technology. vol. 27, no. 13, pp. 2348-2355.
Wang, P., Brambilla, G., Ding, M., Semenova, Y., Wu, Q.,
Farrell, G., 2011. Investigation of single-mode–
multimode–single-mode and single-mode–tapered-
multimode–single-mode fiber structures and their
application for refractive index sensing. J. Opt. Soc.
Am. B. vol. 28, no. 5, pp. 1180-1186.
Wang, Q., Farrell, G., and Yan, W., 2008. Investigation on
single-mode–multimode–single-mode fiber structure.
Journal of Lightwave Technology. vol. 26, no. 5, pp.
512-519.
Winzer, P.J., Essiambre, R.-J., 2006. Advanced optical
modulation formats. Proceedings of the IEEE. vol. 95,
no. 5, pp. 952-985.
Xue, L.-L., Yang, L., 2012. Sensitivity enhancement of RI
sensor based on SMS fiber structure with high
refractive index overlay. Journal of Lightwave
Technology. vol. 30, no. 10, pp. 1463-1469.
Zhu, J.-J., Zhang, A.P., Xia, T.-H., He, S., and Xue, W.,
2010. Fiber-Optic High-Temperature Sensor Based on
Thin-Core Fiber Modal Interferometer, IEEE Sensors
Journal, vol. 10, no. 9, pp. 1415-1418.
DPSKSignalsDemodulationBasedonaGraded-indexMultimodeFiberMismatchSplicedbetweenTwoSingle-mode
Fibers
19