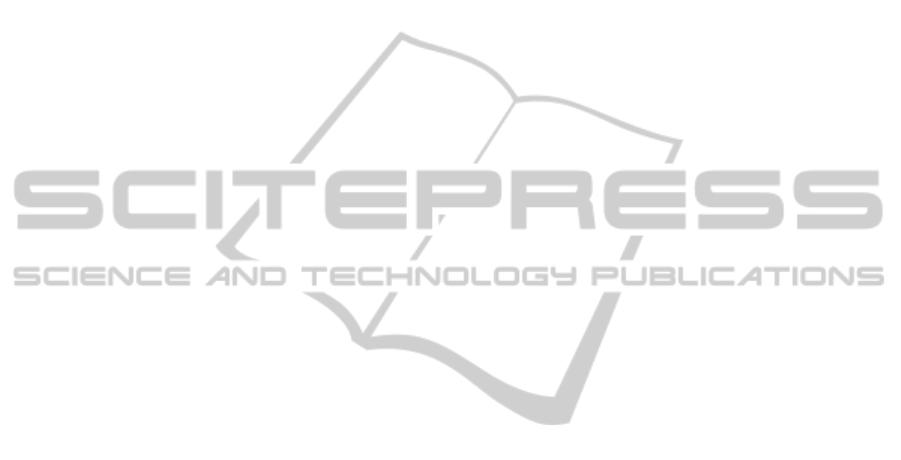
band (Song et. al., 2013). Additionally, the mutual
influence of the resonators is confirmed, when we
analyse the structures with constant
ε
n
. This is
because the peak absorption in those structures is
about ~60%. However, the phase change introduced
by the change of
ε
n
in neighbouring resonators alters
the resonance conditions in each resonator and leads
both to shift of the absorption peaks and high and
broadband absorption.
The ordered structure (with resonators in order:
ε
1
,
ε
2
,
ε
3
,
ε
4
,
ε
5
), similarly as used in (Song et. al.,
2013), may not necessarily be the one with the
widest band width. Probably for this reason, in
(Song et. al., 2013) further optimization of the
structure (individually assorted widths and distances
between particular resonators) was performed to
achieve broad absorption band. This confirms that
the resonators are not independent on each other and
the resulted coupling modes play a role in forming
the broad absorption band. This indicated also, that
the further optimization of our structure by adjusting
separately widths of each space between resonators
may be possible.
5 CONCLUSIONS
A wide-band absorption over 80% was obtained in
the visible range in the periodic metallodielectric
structure with resonators filled with dielectrics of
varied dielectric constants. It occurred that this
device is sensitive to the change of the resonators'
order. The extended broadband absorption in such a
grating structure was attributed to the changed
sequence of those resonators in comparison to the
ordered structure. Those differences indicate that
those resonator are not independent on each other.
Change of the resonators' order allow for lowering
the mismatch of the phase between individual
resonators. The width of the absorption band at
normal incidence is estimated to be ~141 nm, which
is about 6.1 times wider than for a structure with five
identical resonators of
ε
n
=
ε
1
. Moreover, the
bandwidth of the optimized structure shows almost
1.2-fold increase with respect to the absorption
bandwidth of the ordered structure. This work shows
a way to obtain a wideband absorption by
optimizing order of the different resonators in a
grating period. The presented results may appear
useful in detection and imaging at visible
frequencies, angle-selective absorbers,
microbolometers, photodetectors and solar cell
technology.
REFERENCES
Astilean, S., Lalanne, P., Palamaru, M., 2000. Light
transmission through metallic channels much smaller
than the wavelength, Opt. Commun. 175 265.
Bouillard, J.-S., Vilain, S., Dickson, W., Wurtz, G. A.,
Zayats, A. V., 2012. Broadband and broadangle SPP
antennas based on plasmonic crystals with linear chirp,
Scientific Reports 2 829, 1.
Chen, L., Wang, G. P., Gan, Q., Bartoli, F. J., 2010.
Rainbow trapping and releasing by chirped plasmonic
waveguides at visible frequencies, Appl. Phys. Lett. 97
153115.
Diem, M., Koschny, T., Soukoulis, C. M., 2009. Wide-
angle perfect absorber/thermal emitter in the terahertz
regime, Phys. Rev. B 79 033101.
Dolev, I., Volodarsky, M., Porat, G., Arie, A., 2011.
Multiple coupling of surface plasmons in
quasiperiodic gratings, Opt. Lett. 36 1584.
Ferry, V. E., Munday, J. N., Atwater, H. A., 2010. Design
considerations for plasmonic photovoltaics, Adv.
Mater. 22 4794.
Gan, Q., Bartoli, F. J., 2011. Surface dispersion
engineering of planar plasmonic chirped grating for
complete visible rainbow trapping, Appl. Phys. Lett.
98 251103.
Hao, J., Wang, J., Liu, X., Padilla, W. J., Zhou, L., Qiu,
M., 2010. High performance optical absorber based on
a plasmonic metamaterial, Appl. Phys. Lett. 96
251104.
Hu, C., Liu, L., Zhao, Z., Chen, X., Luo, X., 2009. Mixed
plasmons coupling for expanding the bandwidth of
near-perfect absorption at visible frequencies, Opt.
Express 17 16745.
Johnson, P. B., Christy, R. W., 1972. Optical constants of
the noble metals, Phys. Rev. B 6 4370.
Koechlin, C., Bouchon, P., Pardo, F., Jaeck, J., Lafosse,
X., Pelouard, J.-L., Haidar, R., 2011. Total routing and
absorption of photons in dual color plasmonic
antennas, Appl. Phys. Lett. 99 241104.
Kravets, V. G., Neubeck, S., Grigorenko, A. N., 2010.
Plasmonic blackbody: strong absorption of light by
metal nanoparticles embedded in a dielectric matrix,
Phys. Rev. B 81 165401.
Liu, X., Starr, T., Starr, A. F., Padilla, W. J., 2010.
Infrared spatial and frequency selective metamaterial
with near-unity absorbance, Phys. Rev. Lett. 104
207403.
Liu, X., Tyler, T., Starr, T., Starr, A. F., Jokerst, N. M.,
Padilla, W. J., 2011. Taming the blackbody with
infrared metamaterials as selective thermal emitters,
Phys. Rev. Lett. 107 045901.
Maier, S. A., 2007. Plasmonics: Fundamentals and
Applications, New York: Springer.
Meng, L., Zhao, D., Li, Q., Qiu, M., 2013. Polarization-
sensitive perfect absorbers at near-infrared
wavelengths, Opt. Express 21 A111.
Moreau, A., Ciracì, C., Smith, D. R., 2013. Impact of
nonlocal response on metallodielectric multilayers and
optical patch antennas, Phys. Rev. B 87 045401.
BroadbandAbsorptionintheCavityResonatorswithChangedOrder
85