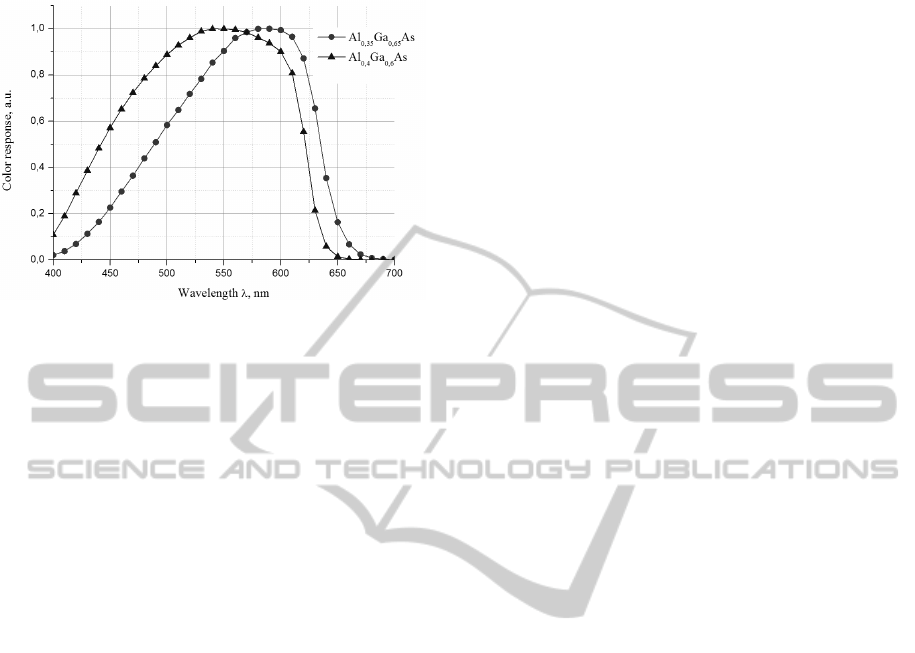
p Al
x
Ga
1-x
As layer was performed.
Figure 5: Photodiodes spectral characteristics.
Calculated spectral characteristics are shifted to the
short-wave range and overall sensitivity increases.
At the same time it could be seen that the greatest
increase in sensitivity at wavelengths less than 0.4
microns takes place not for the widest bandgap
material. Someone can find it strange, but the fact is
that in the more wide bandgap-materials the electron
mobility decreases sharply (from 3.1·10
3
cm
2
/V·s for
Al
0.25
Ga
0.75
As to 5.4·10
2
cm
2
/V·s for
Al
0.419
Ga
0.581
As) for the same surface recombination
rate and lifetime of charge carriers.
Spectral characteristics were measured using a
monochromator MDP and apparatus for measuring
the spectral sensitivity of photodetectors
TTM3.435.088. Figure 5 shows the relative spectral
characteristics of the photodiodes.
It’s seen that, at changing the Al mole fraction by
0.05, maximum of spectral sensitivity was shifted by
40 nm to shorter wavelengths. At the same time the
sensitivity at a wavelength of 475 nm was increased
almost twice by choosing the optimum composition
and thickness of the active p-layer. To achieve
greater sensitivity in shorter wavelengths for
photodiodes antireflection coating on “window”
layer will be used.
Photodetectors created for detecting light from
the scintillator based on AlGaAs/GaAs
heterostructures were produced. Photodetectors
measurements showed the low dark current at the
level of 0.5 nA for phototransistors and 10 nA for
photodiodes, for at the same time high breakdown
voltage was about 600 V and 20 V, accordantly.
Experimentally determined values of
composition and thickness for the Al
x
Ga
1-x
As solid
solution allowed to increase the sensitivity almost
twice from 40 to 75 % at a wavelength 475 nm.
Absolute spectral sensitivity of photodiodes reached
0.13 A/W at λ = 570 nm.
ACKNOWLEDGEMENTS
This study was supported by the Federal Targeted
Program “The development of electronic component
base and radio electronics” for 2008 – 2015, state
contract № 14.430.12.0003.
The work was financially supported Basic part of
State the Federal Targeted Program by the Ministry
of Education and Science of the Russian Federation
within the framework of the federal target program
of Russia in 2014 - 2016 years
.
REFERENCES
Ryzhikov, V., Kozin, D., Grinyov, B., et al. 2003. Nuclear
Instruments and Methods in Physics Research Section
A: Accelerators, Spectrometers, Detectors and
Associated Equipment. v. 505 (1–2) p. 58.
Scintillation detector Patent RF. 2005. 2248588.
Herbert, D. J., D’Ascenzo, N., Belcari, N., et al 2006.
Accelerators, Spectrometers, Detectors and
Associated Equipment, v. 567 (1) p. 356.
Bloser, P. F., Legere, J. S., Bancroft, C. M. et al 2014.
Instruments and Methods in Physics v. 763 (1) p. 26.
Murashev, V. N, Legotin, S. A., Didenko, S. I et al. 2014.
Journal of Alloys and Compounds v. 586 (sup. 1) p.
S553.
Bazalevsky, M. A., Didenko, S. I., Legotin, S. A.,
Rabinovich, O.I., Murashev, V.N., Kazakov, I.P et al
2014. Journal of nano- and electronic physics, v. 6
(3), p. 03019.
Legotin, S. A., Murashov, V. N., Didenko, S. I.,
Rabinovich, O.I. 2014. Journal of nano and electronic
physics. v.6 (3) p. 030201.
Baryshnikov, F. M., Didenko, S. I. Chernykh, A. V.,
Chernykh, S.V. et al. 2012. AOP conference
proceedings ion implantation technology, v. 50 p.
1496.
Koltsov, G. I., Didenko, S. I., Chernykh, A. V., Chernykh,
S.V., et al 2012. Semiconductors, v. 46 (8). p. 1066.
Legotin, S. A., Rabinovich, O. I., Murashov, V. N.,
Didenko S. I., 2014. Nano-and elect. phys. v. 6 (3) p.
03019.
CreatingAlGaAsPhotodetectors
89