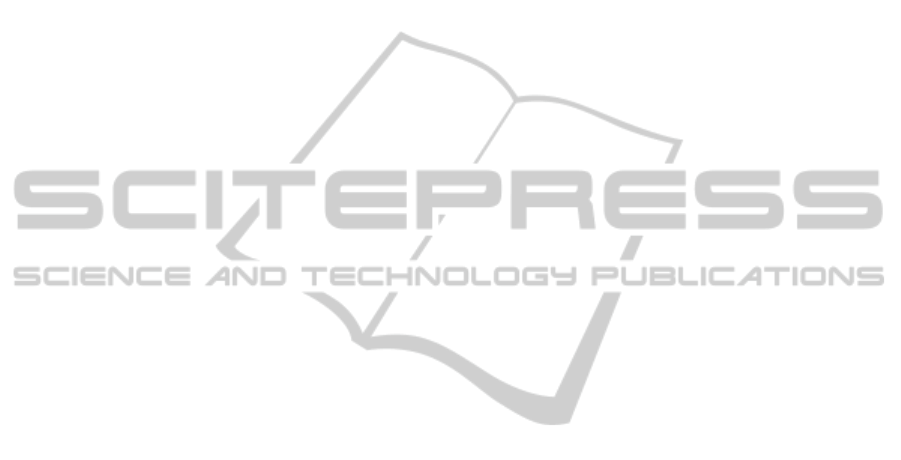
4 SUMMARY
This article describes the characterization of a gated
avalanche photo-detector, used subsequently in a
multi-photon resolving detector. We first experimen-
tally characterize the GAPD and find its efficiency.
We found that the GAPD was susceptible to stochas-
tic resonance, which was affected by temperature and
bias. The stochastic resonance was further investi-
gated, and we saw that it had characteristic frequency
around 66 kHz. When we used gated detection, with
a delay of 1 µs between gate pulses, we were in ef-
fect digitally sampling the stochastic resonance. Dis-
counting these resonances in our experimentally mea-
sured probabilities, we were able to characterize the
GAPD for very low average photon numbers.
A recirculating loop is a simple way of splitting
a Poissonian state into a series of temporal pulses.
We described the statistical properties of such a multi-
photon resolving detector. In our experiments, the re-
circulating loop was set up to achieve a delay of 10µs
between successive optical pulses, while we gated the
avalanche photodiode every 1µs. After each input co-
herent optical pulse we recorded the output for 8 gate
pulses as one byte, and did this for N = 2
24
optical
pulses. We then searched for correlations between
successive gate pulses in the data bytes by looking
at the bit patterns for 3, 4 and 5 bits. The probability
of occurrence of a bit pattern followed the predicted
probabilities, within the noise bounds of the system.
Thus, we conclude that the MPRD could be used for
photon number resolution, for average photon num-
bers as low as n = 0.024 per pulse.
We observed that the stochastic resonance de-
pends on both APD bias and temperature. Conse-
quently, we were able to adjust these parameters and
avoid the resonance, as we set up our photon number
resolving experiments. We believe that we are the first
authors to report on stochastic resonances in a GAPD
system. Further investigations about the origins of the
resonace, along with an appropriate statistical noise
model, will help improve the performance of single
photon detectors.
ACKNOWLEDGEMENTS
This work was partly funded by the Department
of Science and Technology, India under Grant No.
SR/S3/EECE/21/2005. HR and PK were at IIT-
Madras during the course of their studies. HR is also
grateful to IIT-Madras for supporting his work as an
Innovative Student Project.
REFERENCES
Ben-Michael, R., Itzler, M. A., Nyman, B., and Entwistle,
M. (2006). Afterpulsing in InGaAs/InP single photon
avalanche photodetectors. In Dig. LEOS Summer Top.
Meet., pages 15–16, Quebec City. IEEE.
Bennett, C. H., Brassard, G., et al. (1984). Quantum cryp-
tography: Public key distribution and coin tossing. In
Proc. IEEE Int. Conf. Computers, Systems and Signal
Processing, Bangalore, volume 175-179, Bangalore.
New York, IEEE.
Blasej, K., Prochazka, I., and Kodet, J. (2014). Photon
counting detector for high-repetition-rate optical time
transfer providing extremely high data yield. Optical
Engineering, 53:081903.
Bloch, M., McLaughlin, S. W., Merolla, J. M., and Patois,
F. (2007). Frequency-coded quantum key distribution.
Opt. Lett., 32:301–303.
Cova, S., Ghioni, M., Lotito, A., Rech, I., and Zappa, F.
(2004). Evolution and prospects for single-photon
avalanche diodes and quenching circuits. J. Mod. Op-
tic, 51(9-10):1267–1288.
Fitch, M., Jacobs, B., Pittman, T., and Franson, J. (2003).
Photon-number resolution using time-multiplexed
single-photon detectors. Phys. Rev. A, 68(4):043814.
Gammaitoni, L. (1995). Stochastic resonance and the
dithering effect in threshold physical systems. Phys.
Rev. E, 52(5):4691.
Haderka, O., Hamar, M., and Peˇrina Jr, J. (2004). Exper-
imental multi-photon-resolving detector using a sin-
gle avalanche photodiode. Eur. Phys. J. D - Atomic,
Molecular, Optical and Plasma Physics, 28:149–154.
Hadfield, R. H. (2009). Single-photon detectors for opti-
cal quantum information applications. Nat. photonics,
3(12):696–705.
Inoue, K., Waks, E., and Yamamoto, Y. (2003). Differential-
phase-shift quantum key distribution using coherent
light. Phy. Rev. A, 68:022317.
Kolb, K. (2014). Signal-to-noise ratio of geiger-mode
avalanche photodiode single-photon counting detec-
tors. Optical Engineering, 53(8):081904–081904.
Kumar, P., Thevan, S., and Prabhakar, A. (2009). Optimiza-
tion of gated photodetection for quantum key distri-
bution. In SPIE Europe Optics and Optoelec. Conf.,
Prague. SPIE.
Lo, H.-K. and Chau, H. F. (1999). Unconditional security
of quantum key distribution over arbitrarily long dis-
tances. Science, 283(5410):2050–2056.
McDonnell, M. D., Stocks, N. G., Pearce, C. E. M.,
and Abbott, D. (2008). Stochastic resonance: from
suprathreshold stochastic resonance to stochastic sig-
nal quantization. Cambridge University Press, Cam-
bridge.
McIntyre, R. (1966). Multiplication noise in uniform
avalanche diodes. IEEE Trans. Electron Devices, ED-
13(1):164–168.
StochasticResonancesinPhotonNumberResolvingDetectors
45