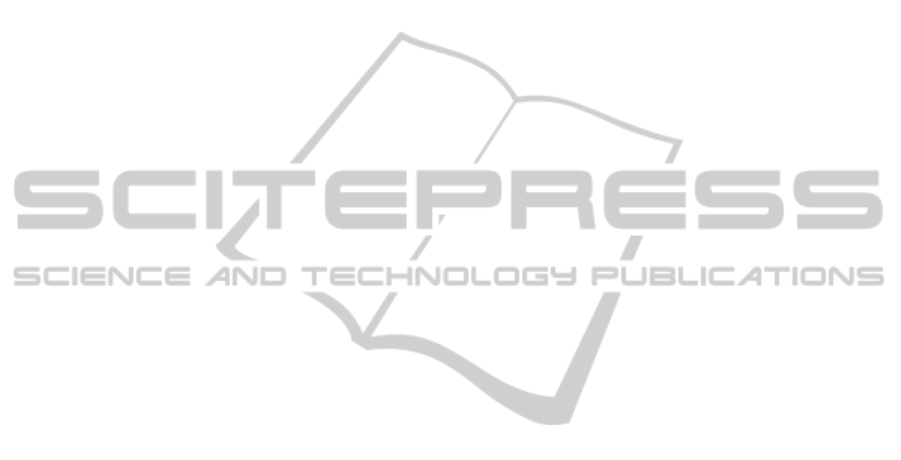
In fact the first adopters, in 1994, found precision
agriculture to be unprofitable. Moreover they found
the instances of implementation of precision
agriculture were few and far between. Further, the
high initial investment in the form of electronic
equipment for sensing and communication meant
that only large farms could afford it. But over the
last decade the advancement in sensing and
communication technologies has significantly
brought down the cost of deployment and running of
a feasible precision agriculture framework. The
development of new electronic low cost sensing
probes (Valente,2006), and the emerging wireless
technologies with low power needs and low data rate
capabilities, which perfectly suites precision
agriculture (Wang, 2006), completely transform this
reality making today precision agriculture possible
to any pocket.
Examples like Montepaldi vineyard where since
2005 a test pilot has been deployed and a
commercial system ”VineSense” developed by
(Davide Di Palma, 2010), or the automated fertilizer
applicator for tree crops developed by (Cugati et
al., 2003) where an input module for GPS and real-
time sensor data acquisition, a decision module for
calculating the optimal quantity and spread pattern
for a fertilizer, and an output module to regulate the
fertilizer application rate. Data communications
among the modules were established using a
Bluetooth network.
USDA research group lead by Evans and
Bergman (Evans, 2007) study a precision irrigation
control of self-propelled, linear-move and center-
pivot irrigation systems. Wireless sensors were used
in the system to assist irrigation scheduling using
combined on-site weather data, remotely sensed data
and grower preferences.
A soil moisture sensor network for monitoring
soil water content changes at high spatial and
temporal scale has develop by the Institute Of
Chemistry And Dynamics Of The Geosphere
(ICDG, 2014).
A wireless infrared thermometer system for in-
field data collection develop by Mahan and Wanjura
(Mahan, 2004) with infrared sensors, programmable
logic controllers and low power radio transceivers to
collect data in the field and transmit it to a remote
receiver outside the field.
4 METHODOLOGY
This PhD work will consider the design and
implementation of a Distributed Sensing System
(DSS) capable of monitoring a wide range of crops
and lands and collecting information such as soil
water content, electrical conductivity (EC - as a
measure of total nutrients), soil thermal properties
(temperature, thermal conductivity, heat capacity,
and thermal diffusivity – as a measure of how
energy is partitioned in the soil profile), water flux,
and environmental temperature at the surface. The
DSS system will use the Multi-functional Heat Pulse
Probe (Valente, 2006), developed by António
Valente. This information will then be sent to and
stored at a central monitoring station. The DSS will
be based on unattended wireless sensors capable of
measuring the parameters of interest, including
aboveground and underground. The DSS needs to be
cost-effective, as less intrusive as possible, and
include a reliable data communications system that
allows data collection from sensors monitoring the
crops, land, and environment. Towards this goal, a
non-intrusive approach is to use low-cost wireless
sensors buried underground, which form a so-called
Wireless Underground Sensor Network (WUSN),
together with a Wireless Aboveground Sensor
Network (WASN) capable of sensing and
transporting data towards the central monitoring
station. In order to fulfill these requirements three
major design options will be considered:
1) the use of commercial off-the-shelf and low-cost
devices;
2) the use of open communications technologies
supporting adaptive modulation-coding
techniques and energy-efficiency mechanisms
and multi-hop routing;
3) a three-tier integrated and open IP-based
communications architecture.
The first-tier will be defined by the Wireless
Underground Sensor Network (WUSN) formed by a
set of nodes (sensor nodes with Multi-Functional
Heat Pulse Sensors), buried underground, which are
connected to one or more aboveground nodes.
Underground sensors may communicate using
technologies such as IEEE 802.11g/n/ah and IEEE
802.15.4/g/m. The use of the 433 MHz and 868
MHz bands will be considered to provide
underground-to-aboveground long range
communications. The underground sensors will send
data to the central monitoring station through the
second-tier.
DistributedSensingSystemModelforFutureInternetAgriculturalApplication
11