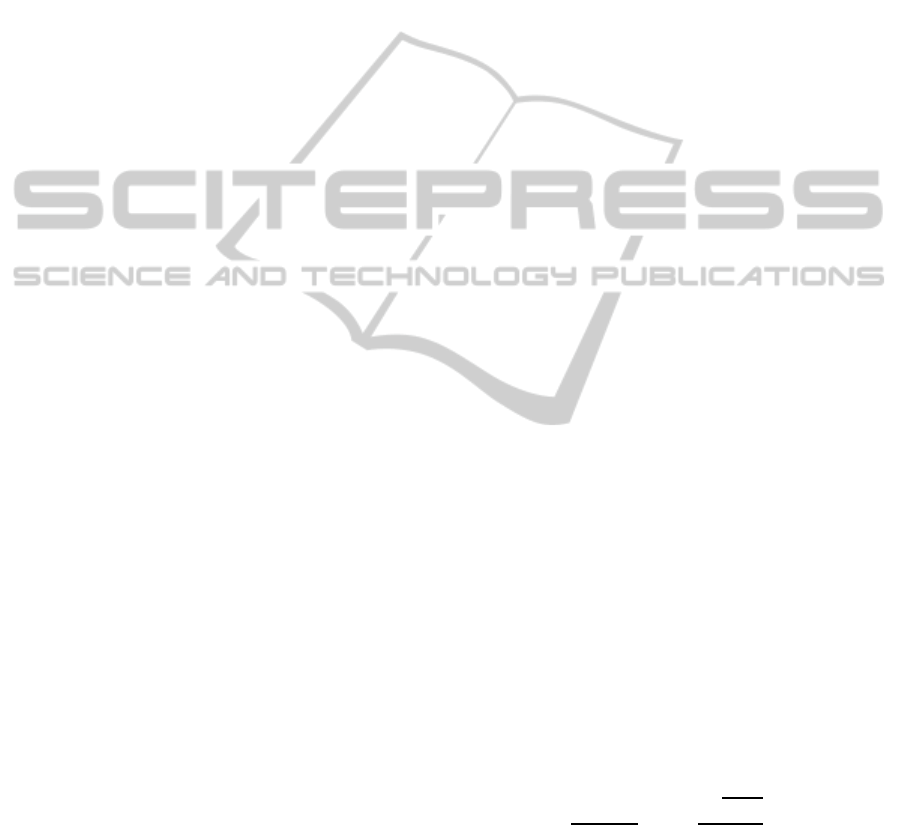
ternary systems As-S-Se and Ge–As–S were prepared
by conventional melt-quenching method from high
purity (5 N) elements in evacuated quartz ampoules
for 8 h at 650
o
C (As based samples) or 1000
o
C (Ge
based samples) in a rocking furnace. Thin-film
samples were prepared by vacuum evaporation of
the bulk glassy materials onto clean glass substrates.
The thermal evaporation process was performed
within a coating system (Tesla Corporation, model
UP-858) at a pressure of about 10
-3
Pa. During the
deposition process the substrates were conveniently
rotated by means of a planetary rotation system to
ensure high homogeneity of the film thickness. The
deposition rate was in the range 1–2 nm/s, measured
continuously using the quartz microbalance technique.
The thickness of the thin films was about 1 m
The laser lithography system DWL 66,
Heidelberg Instruments Mikrotechnik GmbH,
equipped with a vertically polarized continuous-
wave He-Cd laser (=442 nm) was used for the
exposures. The maximal laser power available after
the writing objective to expose the samples was
P
max
=1.8 mW. The laser beam was focused to a spot
diameter of about 640 nm by an objective with a
focal length of 4 mm and a numerical aperture of
0.85. The focus distance of the objective is
controlled by an air gauge based autofocus system.
The applied exposure laser power P was tuned by a
rotable polarizer in steps of 10% from P
max
down to
0.4P
max
, resulting in spot intensities between
5.610
5
W/cm
2
and 2.210
5
W/cm
2
. Structures were
patterned at writing speeds of 25-75 mm/s. An
atomic force microscope (Ultraobjective SIS
attached to an optical microscope) was used to
investigate the morphology of the corrugated surface
of the thin films. The transmission spectra and
diffraction efficiencies were measured with the fiber
coupled spectrometer EPP2000 (StellarNet Inc.)
3 RESULTS AND DISCUSSION
To compare the behavior of thin chalcogenide films
with different optical and thermodynamic properties
under the irradiation with an over band-gap cw laser
a series of single lines was written with different
laser intensities ranging from 2.210
5
to
5.610
5
W/cm
2
on each of the samples of the
different investigated compositions. Created surface
structures were investigated by atomic force
microscopy, and the geometrical profiles of each
line were taken by scanning in the direction
perpendicular to the laser beam movement. The
analysis of such profiles shows that they can be
divided into three main groups (see inset of Fig.1)
indicating various possible formation mechanisms
depending on the laser intensity as well as on the
properties of the distinct thin film.
The curve 1 in the inset of Fig.1 represents a
typical profile for photo-induced expansion that
appears at all used intensities for Ge-As-S thin films
and at low intensity of the writing laser beam for As-
S-Se compositions. In this case the shape of the
corrugated structure follows the Gaussian intensity
profile of the laser beam. The dependences of the
height of the emergent elevations (‘hills’) on the
laser intensity are shown in the upper part of Fig. 1
for different compositions.
The curve 2 in the inset of Fig.1 represents
emerging profiles for the case that a groove with a
simultaneously appearing rim surrounding the center
of the laser spot is created. Formation of such a
profile is typical only for As-S-Se thin films above
an experimentally determined threshold value of
2.810
5
W/cm
2
, whereas for Ge-based ternary
compositions only the creation of grooves without
any rim (see curve 3) was observed. The magnitude
of the groove depth obtained from the AFM images,
is represented in the lower part of Fig. 1 as a
function of exposure intensity for the different
compositions.
As we used an over-bandgap writing laser the
beam energy was totally absorbed within a small
volume of the thin film. The penetration depth d
pd
of
the He-Cd laser beam was evaluated according to
the Beer-Lambert law as d
pd
=1/
(
absorption
coefficient). For As
40
S
60-x
Se
x
compositions d
pd
varies
from 240 nm (x=0) down to 80 nm (x=60) and for
the Ge-based compositions d
pd
is 240 nm almost
independent on the composition. Because of the
extremely high intensity and the total absorption of
the light within a very small volume, the thin
chalcogenide film can be locally and rapidly heated
up to temperatures above T
g
, or even to temperatures
where material decomposition and evaporation take
place. The temperatures on the surface at the center
of a stationary Gaussian heat source for a semi-
infinite solid can be calculated as (Carslaw, 1959):
/
2
∗
,
(1)
where, t – duration of laser interaction with the thin
film, w – waist of the laser beam (0.64 m), K and D
are the thermal conductivity and diffusivity of the
glass substrate (110
-2
W/K*cm and 5.3310
-3
cm
2
/s
respectively), P
a
=P(1-R), where P is the power of
the incident beam and R the reflectivity at the air-
film interface. The potential of this model to
FabricationofSurfaceReliefOpticalElementsinTernaryChalcogenideThinFilmsbyDirectLaserWriting
135