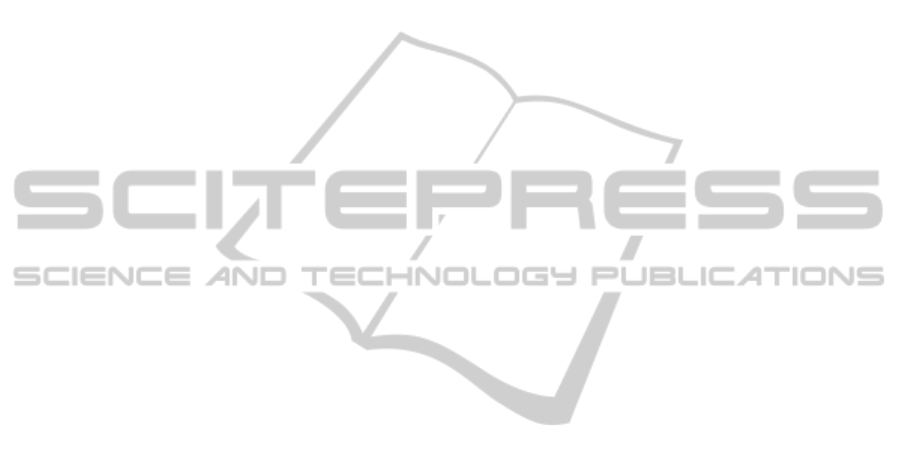
REFERENCES
López-Amo M., López-Higuera J.M., 2011 ‘Fiber Bragg
Grating Sensors: Research Advancements, Industrial
Applications and Market Exploitation’; Bentham
Science Publishers Ltd., Oak Park, Illinois, USA.
López-Higuera, J.M., 1998 Optical Sensors:
Fundamentals, current situation and future trends,
University of Cantabria, Spain.
Kersey, A.D. 1991 Fiber Optic Sensors: An Introduction
for Engineers and Scientists, E. Udd, Wiley, Hoboken,
NJ, USA.
Dandridge A. and Kirkendall C. 2002 Handbook of optical
fibre sensing technology, Chapter 21 John Wiley and
Sons, (, (Baffins Lane, Chichester, UK ).
Abad S., Lopez-Amo M., and Matias I.R., 2002 Handbook
of optical fibre sensing technology, Chapter 22. John
Wiley and Sons, (Baffins Lane, Chichester, UK).
Perez-Herrera, RA, Lopez-Amo, M 2013, ‘Fiber optic
sensor networks’, Invited Papers Optical Fiber
Technology, vol. 19, no. 6, pp 689-699.
Jones, JDC, McBride, R 1988 ‘Multiplexing optical fibre
sensors,’ Optical Fiber Sensors Technology, vol. 2.
Chapman & Hall, London.
Culshaw, B 2004, ‘Optical fiber sensor technologies:
opportunities and – perhaps - pitfalls,’ J. Lightwave
Technol. vol. 22, n. 1, pp. 39-50.
Nakajima, Y, Shindo, Y, Yoshikawa T, 2003 ‘Novel
concept as long-distance transmission FBG sensor
system using distributed Raman amplification’ in
Proc. 16th Int. Conf. Optical Fiber Sensors, Nara,
Japan, pp. 1-4.
Dong, Y, Zhang, H, Chen, L, Bao, X 2012, ‘A 2-cm-
spatial-resolution and 2-km-range Brillouin optical
fiber sensor using a transient differential pulse pair’
Appl. Opt., vol. 51, no. 9, pp. 1229–1235.
Fernandez-Vallejo, M, et al. 2012, ‘46 km long Raman
amplified hybrid double-bus network with point and
distributed Brillouin sensors’, IEEE Sensors Journal,
vol. 12, no. 1, pp. 184-188.
Angulo-Vinuesa, X, et al. 2012, ‘Raman-assisted Brillouin
optical time-domain analysis with sub-meter
resolution over 100 km’, Optics Express, vol. 20, no.
11, pp. 12147–12154.
Angulo-Vinuesa, X, et al., 2012, ‘100km BOTDA
temperature sensor with sub-meter resolution’ in Proc.
of SPIE 22nd International Conference on Optical
Fiber Sensors, vol. 8421, no. 842117.
Soto, MA, Bolognini, G, Pasquale, FD, Thévenaz, L 2010,
‘Long-range Brillouin optical time-domain analysis
sensor employing pulse coding techniques’ Meas. Sci.
Technol., vol. 21, no. 9, pp 094024.
Song, KY, Yang, S 2010, ‘Simplified Brillouin optical
time-domain sensor based on direct modulation of a
laser diode’ Opt. Express, vol. 18, no. 23, pp. 24012-
24018.
Nguyen, D.M., et al. 2012, ‘Sensitivity enhancement in
long-range distributed Brillouin fiber sensor using an
anti-Stokes single-sideband probe and a bidirectional
EDFA’ Photonics Global Conference, article
no.6458002.
Soto, MA, et al. 2012, ‘Simplex-Coded BOTDA Sensor
over 120-km SMF with 1-m Spatial Resolution
Assisted by Optimized Bidirectional Raman
Amplification’ IEEE Photon. Technol. Lett. vol. 24,
no. 20 pp. 1823-1826.
Taki, M, Soto, MA, et al. 2011, ‘Long-range BOTDA
sensing using optical pulse coding and single source
bi-directional distributed Raman amplification’, in
proc. of IEEE Sensors 10th, no 6127160, pp. 382–385.
Bernini, R, Minardo, A, Zeni, L 2011, ‘Long-range
distributed Brillouin fiber sensors by use of an
unbalanced double sideband probe’ Opt. Express, vol.
19, no. 24, pp. 23845–23856.
Zhou, DP, Li, W, Chen, L, Bao, X 2013, ‘Distributed
Temperature and Strain Discrimination with
Stimulated Brillouin Scattering and Rayleigh
Backscatter in an Optical Fiber’ Sensors vol. 13, no. 2
pp. 1836-1845.
Mao, Y, Guo, N, Yu, KL, Tam, HY 2012, ‘1-cm-Spatial-
Resolution Brillouin Optical Time-Domain Analysis
Based on Bright Pulse Brillouin Gain and
Complementary Code’ IEEE Photonics Journal, vol.
4, no. 6, pp. 2243-2248.
J. Urricelqui, A. Zornoza, M. Sagues, A. Loayssa, 2012
‘Dynamic BOTDA measurements using Brillouin
phase-shift,’ Proc of SPIE 22nd International Conf. on
Optical Fiber Sensors, vol. 8421, No. 842125.
Yamashita, RK, He, Z, Hotate, K 2012, ‘Spatial resolution
improvement based on intensity modulation in
measurement of Brillouin dynamic grating localized
by correlation domain technique,’ Proc. of SPIE
OFS22, vol. 8421, No. 84219H, 2012.
S. Delepine-Lesoille, X. Phéron, J. Bertrand, et al. 2012,
‘Industrial Qualification Process for Optical Fibers
Distributed Strain and Temperature Sensing in Nuclear
Waste Repositories’ Journal of Sensors, vol. 2012, no.
369375, 9 pages.
Bernini, R, Minardo, A, Zeni, L 2011, ‘Lecture notes in
electrical engineering,’ 16
th
Conference on Italian
Association of Sensors and Microsystems, vol. 109,
pp. 235-239.
Fry, ES 2012 ‘Remote sensing of sound speed in the ocean
via Brillouin scattering’ in: Proc. of SPIE, vol. 8372,
no. 837207.
Fu, HY, et al. 2008, ‘A novel fiber Bragg grating sensor
configuration for long-distance quasi distributed
measurement’, IEEE Sensors Journal, vol. 8, no. 9,
pp. 1598-1602.
Saitoh, T, Nakamura, et al. 2007, ‘Ultra-Long-Distance
Fiber Bragg Grating Sensor System’ IEEE Photon.
Technol. Lett., vol. 19, no. 20, pp. 1616–1618.
Saitoh, T, Nakamura, K, et al. 2008, ‘Ultra-long-distance
(230 km) FBG sensor system,’ in: Proc. SPIE, vol.
7004, pp. 70046C-4.
Leandro, D, et al. 2011, ‘Remote (155 km) Fiber Bragg
Grating Interrogation Technique Combining Raman,
Brillouin, and Erbium Gain in a Fiber Laser’, IEEE
Photon. Technol. Lett., vol. 23, no. 10, pp. 621–623.
FiberOpticSensorConfigurations
145