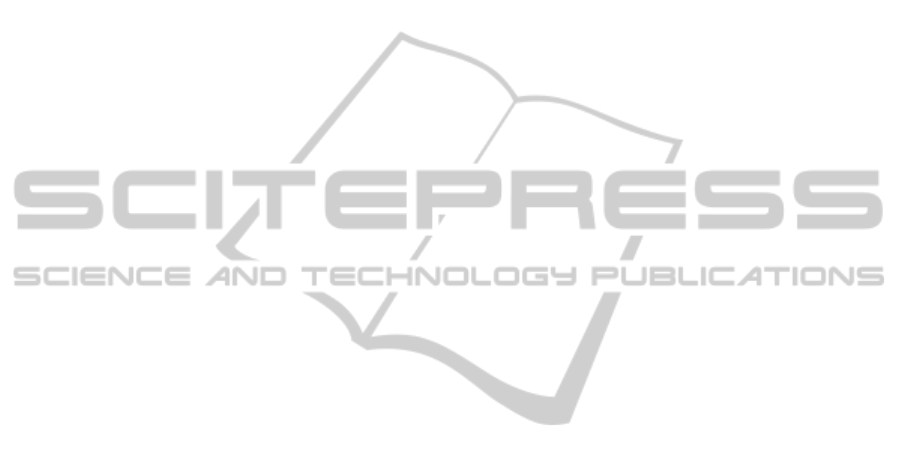
multi-axial design equipped with a precise diagnostic
system of laser beam path deformation, in order to
stabilize the scale factor k
S
better than 10
−10
.
Recent studies and experimental activities made in
this context on the middle-size heterolitic RL ’G-Pisa’
(Belfi J. et al., 2012), have motivate the design of the
GINGER device. It consists in a triaxial system of
large square heterolitic RLs arranged in a octahedral
structure (figure 1). A suitable location for this device
could be the underground facility of LNGS (Labora-
tori Nazionali del Gran Sasso - Italy).
To accomplish GINGER’s goal needs a control of the
systematic errors related to the fluctuation of the cav-
ity geometry and the laser active medium parameters.
As regards the last issue, a set of spectroscopic di-
agnostic of the active medium parameters and an off-
line denoising method that, based on the Kalman filter
approach, subtracts from the raw Sagnac data the sys-
tematic effects induced by the non-linearity of the RL
dynamics, has been developed (Beghi A. et al., 2012)
(Cuccato D. et al., 2014). In the following, we’ll dis-
cuss mainly about the first research field.
4 RING CAVITY GEOMETRY
CONTROL
The measure of Lense-Thirring effect in a ground-
based laboratory requires a stabilization of the scale
factor k
S
better than 10
−10
. This implies an accuracy
on mirror positions better than 1 nm and on the ring
cavities relative orientation better than 1 nrad.
In a square RL, a so strict control on mirror posi-
tions can be reduced if the absolute length of the diag-
onal cavities is stabilized in addition to the perimeter
one, so that the closed optical path shape is that of a
regular square. Our theoretical and numerical stud-
ies are reported in detail in Ref.(Santagata R. et al.,
2014). We showed that if the length of the two diago-
nals are locked to the same value, the perturbations to
the mirror positions affect only quadratically the ring
laser scale factor. This constraint reduces the mirror
position fluctuation at a level of 1 part in 10
10
, even if
the two lengths are stabilized to values that differs at
a micrometric scale.
These results motivated the design of GP2 (Di
Virgilio A. et al., 2014), an intermediate prototype
of GINGER specifically devoted to test the active
control strategies and, in particular, to implement
the length stabilization of the diagonal resonators by
means of optical interferometry. In addition to it, the
GINGERino prototype has also been developed last
year (Di Virgilio A. et al., 2014); it is especially birth
to analyze the seismic noise at LNGS location, but
also to tryout the SNR improvement of a larger cav-
ity.
The optical setup of both devices consists in four
supermirrors each one contained in a steel holder
placed at the corner of a granite support fixed on a
concrete base. Steel pipes connected by the mirror
holders define the vacuum chamber that encloses the
optical path of the circulating beams along a square
loop. The vacuum chamber is filled with a mixture of
He-Ne and the capacitive discharge for laser excita-
tion, consisting in a pyrex capillary, is located in the
middle of a side of the cavity. The laser frequency is
stabilized with respect an optical frequency reference
in order to actively control the ring cavity perimeter.
In section 5 GP2 and GINGERino prototype are
described. To stabilize the absolute length of di-
agonal resonators we worked out an interferometric
metrology technique and we tested it on two Fabry-
Perot cavities simulating the ring diagonals on an op-
tical bench. We report some details about this work,
whose results has been published in Ref.(Belfi J. et
al., 2014), in section 6. Finally, in section 7 expected
outcome and future perspectives are discussed.
5 METHODOLOGY
5.1 GP2
5.1.1 Optical Setup
GP2 is the seed device for the next generation het-
erolitic active-stabilized RLs. It has been designed in
order to gain a long term stability and accuracy of the
scale factor, via a precise control of the systematic er-
rors related to the fluctuation of the cavity geometry
and the active medium parameters. In particular, it is
dedicated to implement a length stabilization of the
diagonal cavities using optical interferometric tech-
niques.
Figure 2 shows a drawing of GP2 (above) and its
installation in a clean room at INFN Pisa laboratories
in March 2014 (below). The granite slab whereon the
cavity is placed is oriented along the local latitude in
order to maximize the Sagnac signal and minimize
the orientation errors on scale factor. The four mir-
rors holders are placed at the corner of a square gran-
ite slab and the vacuum chamber encloses the beam
optical path along a square loop 1.60 m length in
side. Figure 3 shows a preliminary Sagnac spectrum;
a Sagnac frequency of 184 Hz has been observed, as
expected.
To check the quality factor Q = 2π fτ of the laser
PHOTOPTICS2015-DoctoralConsortium
12