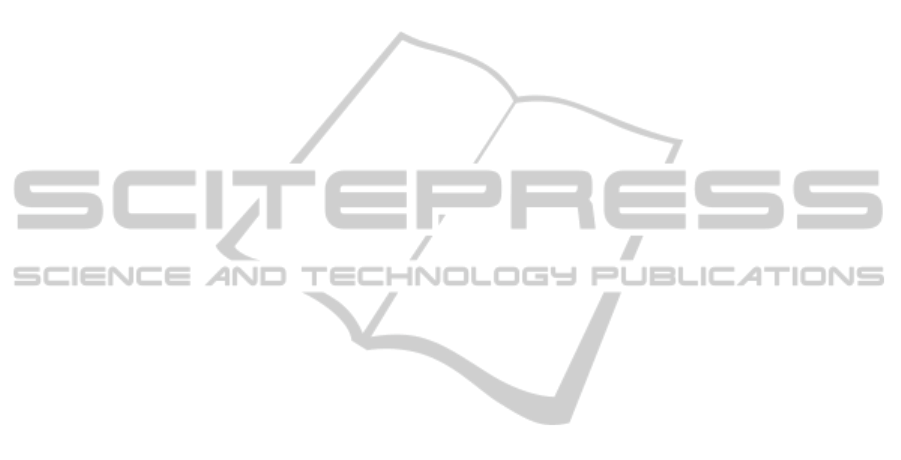
2 OUTLINE OF OBJECTIVES
The objectives of the presented work are focused on
addressing the major issues faced by a-SNOM
techniques. Thus, an advancement regarding the
mathematical models associated with the physical
phenomena is on the objective list. Analyses of the
mathematical models will provide clues for
improvements with respect to data interpretation
process – that is another major objective. Because of
the difficulties associated with a-SNOM techniques,
few investigation areas had been explored till now.
Thus, an increased attention is given to new research
areas where a-SNOM can be used successfully – this
being the third major objective of the presented
work.
3 STATE OF THE ART
In the frame of near-field optical investigations, two
major categories of microscopes are available: (a)
aperture-SNOM, and (b) apertureless-SNOM. The
first class uses a metal-coated tapered optical fibre
as a probe (NOVOTNY et al., 1995). Although
SNOM with aperture is more popular and already
commercially available, it suffers from a major
drawback, as a compromise is necessary between the
dimension of the aperture and the power of the
incident laser beam. For higher resolution, the
aperture needs to be smaller; in the same time, for an
efficient transmission, a smaller aperture requires a
greater optical power, which may be harmful to the
probe’s tip. This situation limits the lateral
resolution to about 100 nm.
The second class of near-field microscopes is
represented by the so-called “apertureless-SNOM”.
Its functioning principle has been already presented
in the introductive part of the paper. As this class of
near-field microscopes present several advantages
over SNOM with aperture (better resolution;
independence on the radiation wavelength), it itself
confronts with a series of difficulties. The major
drawback is the presence of a background light
(noise), which affects the detection process. Several
techniques have been developed to date in order to
discriminate between the near-field signal and the
signal generated by the background light. Higher
harmonic demodulation (HHD) (Maghelli et al.,
2001) takes advantage over the nonlinear
dependence of the intensity of the light emitted from
the near-field with the distance between the tip and
the sample’s surface. Thus, oscillating the probe in a
sinusoidal movement above the sample (as in AFM
tapping mode) and detecting the signal on a higher
harmonic of the oscillation frequency f
o
, the signal-
to-noise ratio (SNR) will increase. This is due to the
rather linear dependency of the background light on
the tip-sample distance.
However, HHD is not sufficient for efficient
background reduction. Along with it, interferometric
detection is also used. During the last decade, three
major interferometric methods were used: homodyne
(Knoll and Keilmann, 2000), heterodyne (Gomez et
al., 2006) and pseudoheterodyne detection (Ocelic et
al., 2006). Among these, the last one proved to be
particularly efficient and easy to implement. Is
consists in a modified Michelson interferometer, in
which one arm is used for the illumination of the tip
and for collection of the near-field emitted light
(together with the background). The other arm is
travelled by a reference beam, which is phase-
modulated by an oscillating mirror. This reference
mirror oscillates with a certain frequency M (much
smaller than the oscillating frequency of the probe)
and certain amplitude, A. The two beams finally
interfere on the detection path, on which a detector
is placed. Without the reference beam, the Fourier
spectrum of the electric signal generated by the
detector will contain signal components situated at
the probe’s oscillation frequency and at its
harmonics. When the reference beam is present, the
phase modulation determines the appearance of two
side-bands around each initial harmonic component.
These side-bands contain components situated at
nf
o
±mM. It had been demonstrated that the
background light has a minimal influence on these
side-bands; thus, detection on a frequencies situated
in these side-bands assures for best SNR.
A recently reported alternative to pseudo-
heterodyne detection is represented by the
combination between synthetic optical holography
and s-SNOM (Schnell et al., 2014). In this case, the
resulting image is a hologram, which contains both
amplitude and phase data of the near-field emitted
light.
Regarding the mathematical models, two major
models are largely used: Oscillating Point Dipole
Model (OPDM) (Knoll and Keilmann, 1999) and
Finite Dipole Model (FDM) (Cvitkovic et al., 2007).
To date, a-SNOM has been applied successfully in
several areas, including: nano-imaging (Schnell et
al., 2010), characterisation of plasmonic structures
(Kim and Kim, 2012), near-field spectroscopy
(Stiegler et al., 2011), nano-chemical
characterisation (Berweger et al., 2013), or SHG and
fluorescence on representative samples (Huang et
PHOTOPTICS2015-DoctoralConsortium
20