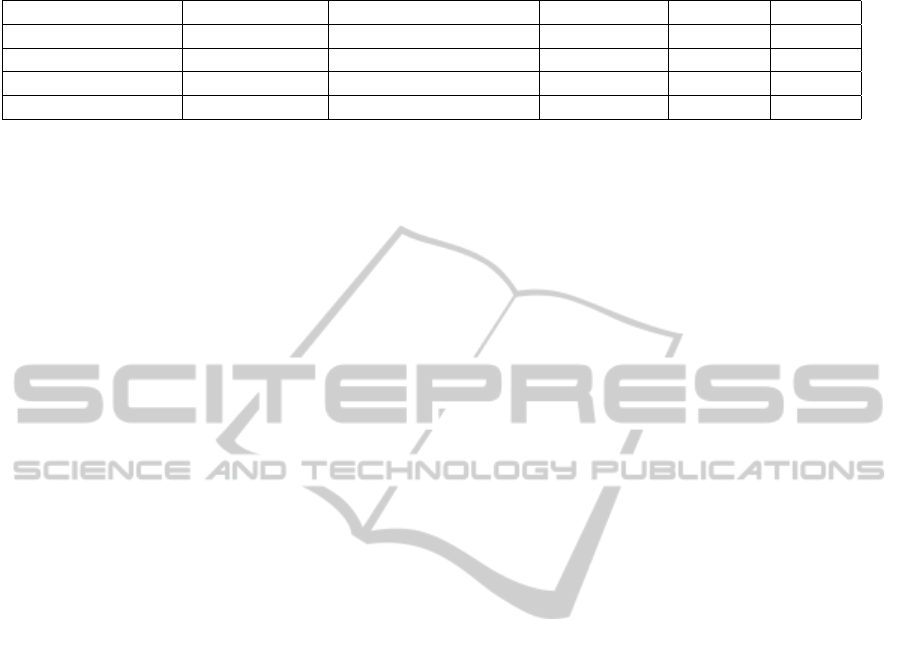
Table 1: Characteristics of the fabricated SOH slot-waveguide ring resonator resonators.
circumference [µm] slot width [nm] center wavelength [nm] FWHM [nm] FSR [nm] Q-factor
69.3 130 1598.03 0.31465 6.7 5079
69.3 150 1586.5 0.47424 7 3345
95.5 130 1597.05 0.05497 3.3 29054
95.5 110 1573.58 0,01567 3.4 100420
6 STAGE OF THE RESEARCH
Three year of research will be needed. The first year
has been completed successfully. This period in-
cluded a first concept development and optimization
through numerical simulations as well as a first de-
sign realization. Figure of merits such as field con-
finement factors and nonlinear effective areas of slot-
waveguide structures are calculated and presented at
the EOSAM 2014 in Berlin as an oral presentation
(Steglich et al., 2014). Further simulation results
and SOI slot-waveguide design trade-offs will be pre-
sented at the PHOTOPTICS 2015 in Berlin as FULL
paper with an oral presentation (Steglich et al., 2015a)
and at the CLEO/Europe 2015 in Munich (Steglich
et al., 2015b). Beside theoretical investigations, first
electro-optical modulators have been fabricated with
a SiGe BiCMOS pilot line at the IHP. We expect to
demonstrate our first measurements on SOH modula-
tor during the next few months.
ACKNOWLEDGEMENTS
The authors would like to thank the German Federal
Ministry of Education and Research (BMBF) for the
financial support under contract no. 03FH086PX2,
the University of Applied Sciences Wildau (THWi),
Germany, and the Ministry of Science, Technology
and Culture of the federal state Brandenburg, Ger-
many, for financial support. The authors would also
like to thank Fr. Nanni, P. Prosposito, F. De Matteis,
and R. De Angelis from the University of Rome Tor
Vergata, Italy, St. Meister and A. Al-Saadi from the
Technical University Berlin, Germany, and L. Zim-
mermann, D. Knoll, D. Stolarek, J. Katzer, St. Lis-
chke, H. Silz, B. Tillack and W. Mehr from the In-
stitute of High-Performance Microelectronics (IHP),
Germany, for their encouragement and support in the
framework of the Joint-Lab IHP-THWi.
REFERENCES
Agrawal, G. (2012). Nonlinear Fiber Optics. Academic
Press, 5 edition.
Almeida, V. R., Xu, Q., Barrios, C. A., and Lipson, M.
(2004). Guiding and confining light in void nanos-
tructure. Opt. Lett., 29(11):1209–1211.
Barrios, C. A., Gylfason, K. B., S
´
anchez, B., Griol,
A., Sohlstr
¨
om, H., Holgado, M., and Casquel, R.
(2007). Slot-waveguide biochemical sensor. Opt.
Lett., 32(21):3080–3082.
Claes, T., Molera, J., De Vos, K., Schachtb, E., Baets, R.,
and Bienstman, P. (2009). Label-free biosensing with
a slot-waveguide-based ring resonator in silicon on in-
sulator. Photonics Journal, IEEE, 1(3):197–204.
Dalton, L. R., Sullivan, P. A., and Bale, D. H. (2010). Elec-
tric field poled organic electro-optic materials: State
of the art and future prospects. Chemical Reviews,
110(1):25–55.
Dell’Olio, F. and Passaro, V. M. (2007). Optical sensing
by optimized silicon slot waveguides. Opt. Express,
15(8):4977–4993.
Feng, N.-N., Michel, J., and Kimerling, L. (2006). Optical
field concentration in low-index waveguides. Quan-
tum Electronics, IEEE Journal of, 42(9):885–890.
Gould, M., Baehr-Jones, T., Ding, R., Huang, S., Luo,
J., Jen, A. K.-Y., Fedeli, J.-M., Fournier, M., and
Hochberg, M. (2011). Silicon-polymer hybrid slot
waveguide ring-resonator modulator. Opt. Express,
19(5):3952–3961.
Koos, C., Jacome, L., Poulton, C., Leuthold, J., and Freude,
W. (2007). Nonlinear silicon-on-insulator waveg-
uides for all-optical signal processing. Opt. Express,
15(10):5976–5990.
Korn, D., Jazbinsek, M., Palmer, R., Baier, M., Alloatti, L.,
Yu, H., Bogaerts, W., Lepage, G., Verheyen, P., Absil,
P., Guenter, P., Koos, C., Freude, W., and Leuthold,
J. (2014). Electro-optic organic crystal silicon high-
speed modulator. Photonics Journal, IEEE, 6(2):1–9.
Korn, D., Palmer, R., Yu, H., Schindler, P. C., Alloatti,
L., Baier, M., Schmogrow, R., Bogaerts, W., Sel-
varaja, S. K., Lepage, G., Pantouvaki, M., Wouters,
J. M., Verheyen, P., Campenhout, J. V., Chen, B.,
Baets, R., Absil, P., Dinu, R., Koos, C., Freude,
W., and Leuthold, J. (2013). Silicon-organic hybrid
(soh) iq modulator using the linear electro-optic effect
for transmitting 16qam at 112 gbit/s. Opt. Express,
21(11):13219–13227.
Leuthold, J., Koos, C., Freude, W., Alloatti, L., Palmer, R.,
Korn, D., Pfeifle, J., Lauermann, M., Dinu, R., Wehrli,
PHOTOPTICS2015-DoctoralConsortium
60