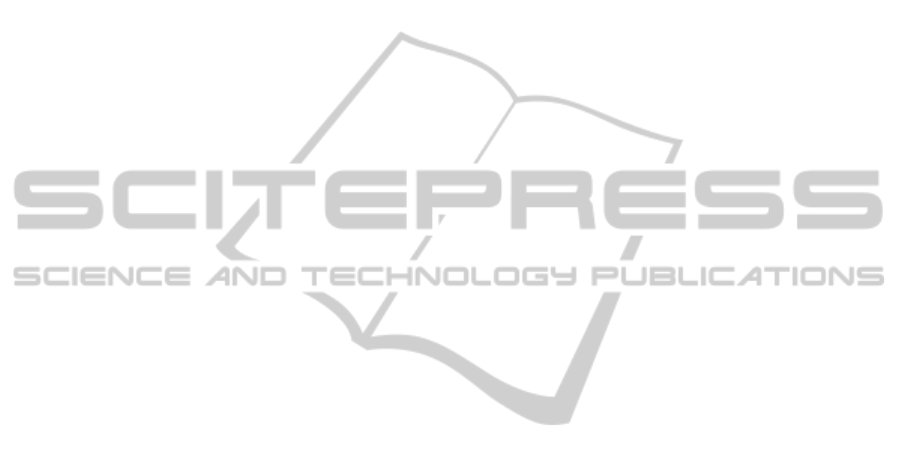
be reduced by 24.24%. Cost are either caused by
buying electricity from the grid or by burning gas for
the CHP. With PV integrated in house 1, less
electricity has to be purchased from the grid. Since
the CHP generates the same amount of heat during
the day in the separated and the combined cases, the
effect of cost reduction is only caused by the
enhanced integration of PV.
5 CONCLUSIONS AND
OUTLOOK
The results of the three simulations have illustrated a
number of benefits of coupling the heat and electricity
systems of domestic buildings. House 1 results show
the benefits of having both electrical and thermal
storage to support a CHP plant by maximising its
utilisation. House 2 showed the advantage of using a
heat pump to better utilise the PV output. Finally the
combined system shows the further benefits of the
synergy by illustrating that greater benefits can be
gained by optimising on a larger scale. The utilisation
of PV increased, greatly reducing the cost of energy
for both households.
Many simplifications have been made in this
study, for example regarding the efficiency and linear
operation of the energy storage characteristics, and
constant efficiency characteristics for the CHP and
heat pump. A better quantification of the advantages
can be gained by more detailed modelling of the
various components. This will result in non-linear
system dynamics, and therefore more sophisticated
optimisation techniques will be required.
Furthermore the results of this study suggest that
it is worth optimising several domestic buildings or
households together. This will also allow other
technologies to be investigated such as CHP plants
for entire building blocks.
These two components, more detailed modelling,
and a wider scope of households, will guide the
further work. As mentioned the wider project is to
develop a framework for optimising energy systems
at a city district or building block level. Another
important factor that this study has not considered is
the costing of various systems. Correct costing can
allow the modelling framework developed in this
paper to be extended and used for calculations such
as optimal sizing of energy storage units and PV
installations.
Besides sizing, a modelling framework will also
allow for the evaluation of more sophisticated control
techniques within a smart grid framework.
Particularly the use of online control, where system
measurements are used to re-optimise the operation at
every time step has shown to bring cost benefits.
REFERENCES
Brenna, M., Falvo, M.C., Foiadelli, F., Martirano, L.,
Massaro, F., Poli, D., Vaccaro, A., 2012. Challenges in
Energy Systems for the Smart Cities of the Future. In
Energy Conference and Exhibition (ENERGYCON),
Florence, p. 755-762.
Brunner, M., Schäfer, I., Rudion, K., Tenbohlen, S., 2014.
Impacts of different seasons on the contribution of heat
pumps to voltage stability. In VDE-Congress 2014
Smart Cities Proceedings, Frankfurt am Main, no. 46.
Geidl, M., Koeppel, G. Favre-Perrod, P., Klockl, B.,
Andersson, G., Frohlich, K ., 2007. Energy hubs for the
future. In IEEE Power and Energy Magazine, vol. 5,
no. 1, pp. 24–30.
Giuntoli, M., Poli, D., 2013. Optimized Thermal and
Electrical Scheduling of a Large Scale Virtual Power
Plant in the Presence of Energy Storages. IEEE
Transactions on Smart Grid. vol. 4, no. 2, p. 942-955.
Hemmes, K., Zachariah-Wolf, J.L., Geidl, M., Andersson,
G., 2007. Towards multi-source multi-product energy
systems, International Journal of Hydrogen Energy,
Vol 32, Issues 10–11, p. 1332-1338.
Houwing, M., Negenborn, R.R., De Schutter, B., 2011.
Demand Response With Micro-CHP Systems.
Proceedings of the IEEE, 99(1), 200-213.
Quaschning, V., 2013. Regenerative Energiesysteme,
Hanser Verlag. München, 8
th
edition.
Vanhoudt, D., Geysen, D., Claessens, B., Leemans, F.,
Jespers, L., Van Bael, J., 2014. An actively controlled
residential heat pump: Potential on peak shaving and
maximization of self-consumption of renewable
energy, Renewable Energy, vol. 63, p. 531-543.
VDI 4655, 2008. Reference load profiles of single-family
and multi-family houses for the use of CHP systems.
Beuth Verlag, Berlin.
ElectricityandHeatSectorCouplingforDomesticEnergySystems-BenefitsofIntegratedEnergySystemModelling
71