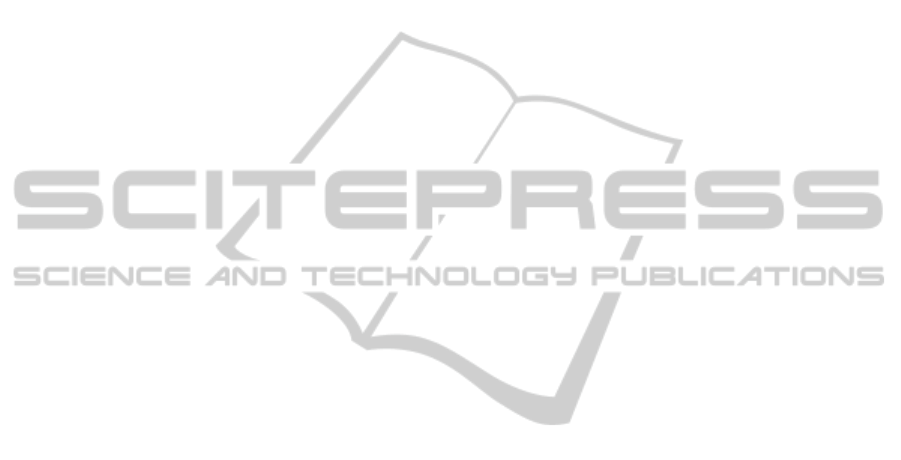
efficiency. Urban energy systems have been defined
as the combined process of acquiring and using
energy to satisfy the demands of a given urban area
(Keirstead, 2013).
Latest advancements of ICTs have made possible
today to design and operate in a optimized way
individual energy distribution networks, which
include smart electricity grids, district
heating/cooling networks, gas grids, and beyond-
energy resource distribution networks, like ICT,
transport /fueling networks.
The state of the art for Infrastructure integration
implementation is for:
Different limited-scale pilot applications have
been implemented all over Europe (if not world)
No synergies in place at any level among the
different functional domains or infrastructures,
which is the main reason for:
Ongoing applications are tailored to
optimize resource efficiency in a single
domain (i.e. either energy, either
transport/mobility, either water, …)
Energy and resource-efficient district
planning and retrofitting are very seldom
cost-effective and very rarely citizens are
fully engaged in decision making process
Municipalities look at infrastructure networks as
individual and unrelated systems, producing
resource usage inefficiencies
Governance is based on unconstrained growing
demand results in inefficient and unsustainable
Current design and operation do not integrate
end users, in terms of the heterogeneity of their
energy and other resources demand and
behaviors (e.g. car ownership as unique
transport mode) and their crucial role in
selecting and using technological options (e.g.
selection and appropriate operation of energy
efficient technologies);
Disjoint and parallel delivery of different
infrastructure streams prohibits the development
of potential joint solutions (e.g. co-treatment of
waste and wastewater), or even substitutions
(e.g. substitution of electricity with gas through
micro-combined heat and power (CHP)),
between infrastructure systems. These
characteristics represent major barriers to
technical innovation and longer term
sustainability.
However the urban landscape nowadays is
rapidly moving forward towards an overall
sustainable system with embedded local energy
generation from Renewable Energy Sources (power
from PV, thermal energy from solar collectors) to be
exploited, used and/or integrated in whatever form
in the energy distribution networks aimed at
reducing energy intensity of human activities and
increase the smart city energy efficiency. The
emerging paradigm is for a constrained demand, in
which the basic assumption is the “limited natural
resources availability”, which need to be exploited at
largest possible extent when and where they are
available and suitably managed.
Emerging ecological models represent cities as
living self-sustainable organisms which pass trough
different life stages, each of them with different
level of energy consumption (and materials) to carry
out human processes (Urban Metabolism).
Leveraging on urban metabolism sustainable
models, cities may take on a key role in nurturing an
innovative smart sustainable development model,
aimed at activating a circular economy through a
synergistic approach, combining the city economic,
logistic and industrial activities with citizens quality
of life and overall energy efficiency and carbon
footprint reduction.
Innovation can be stimulated by regarding cities
as living organisms, with the continuous flow of
inputs and outputs as their “metabolism.” More
circular urban metabolism that treats outputs from
one use as inputs to another would help cities
increase resource productivity and adapt to a future
of resource limitations and climate uncertainty.
Self-sustainability for cities means a “system-of-
system” vision in which the objective is to consume
locally generated resources as much as possible,
with a view to reduce the energy cost necessary for
transporting locally generated resources elsewhere.
As such, different synergies may be found within
energy consuming (transport, ICT) and energy
transporting infrastructures (smart energy networks)
stakeholders, ranging from planning stage (Co-
Planning of a district-level decentralized CHP
generation system to couple heat and power system),
to the construction (es. digging sharing for telco and
power networks), operation phase (es. sharing assets
and facility among street lighting, telco networks,
information access and electric vehicle recharging,
billing IT systems sharing, coupled optimized
operation) and at level of service delivery and
business model (integrated services built upon data-
level cross-resource interoperability, without any
operational optimized multi-resource management,
multi-utility value proposition like providing the
requested thermal comfort level to the households).
Despite there have been a number of attempts for
designing and operating multi-carrier systems, the
large majority of these approaches are limited to
SMARTGREENS2015-4thInternationalConferenceonSmartCitiesandGreenICTSystems
108