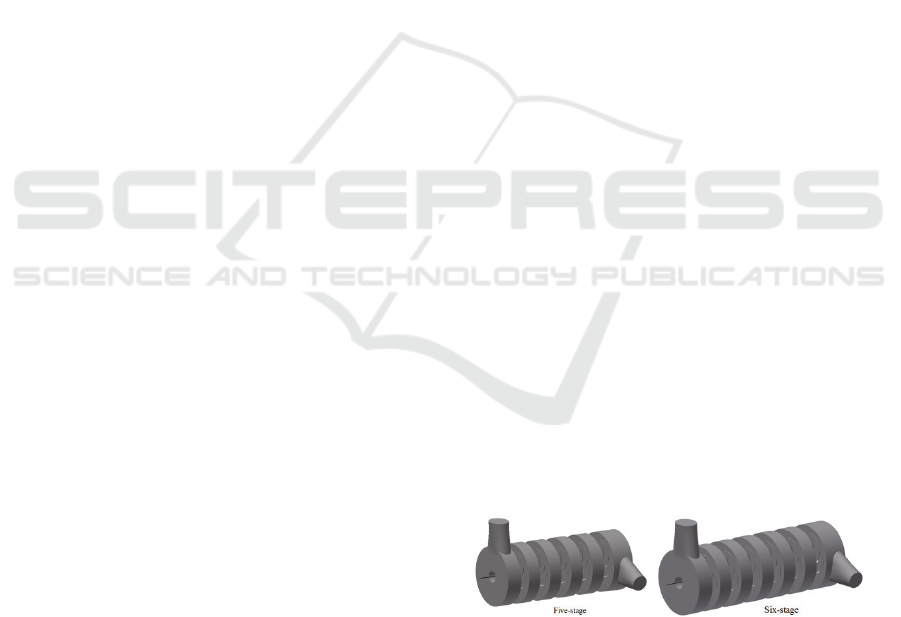
Parametric Study of Liquid Flow in Five- and Six-stage Centrifugal
Pumps
Lahbib Kerbouci and Guyh Dituba Ngoma
University of Quebec in Abitibi-Témiscamingue, School of Engineering’s Department, 445, Boulevard de l’Université,
Rouyn-Noranda, Quebec, J9X 5E4, Canada
Keywords: Multistage Centrifugal Pump, Impeller, Diffuser, Computational Fluid Dynamics (CFD), Modeling and
Simulation.
Abstract: Two models of a multistage centrifugal pump using a five- and six-stage centrifugal pumps were developed
and numerically investigated. The continuity and Navier-Stokes equations with the k- turbulence model
and standard wall functions were used by means of the ANSYS-CFX code. To enhance the design of the
multistage pump, the concept consisting of varying three parameters at a time was used. Thus, the combined
effects of the impeller blade angle, the impeller blade number and the impeller blade width on the
performance of the five- and six-stage centrifugal pumps was analyzed. The results obtained reveal, among
other things, that the highest pump efficiency was reached for the outlet impeller blade angle of 25.38°, the
number of impeller blades of 7, and the impeller blade width of 7 mm.
1 INTRODUCTION
Multistage centrifugal pumps are widely used in
industrial and mining enterprises (Gülich, 2010). For
a more performing multistage pump, its design
parameters, such as the number of stages, impeller
blades, diffuser vanes and diffuser return vanes,
angle of the impeller blade, height of the impeller
blade and diffuser vane, the width of the impeller
blade and diffuser vane, the impeller and diffuser
diameter, the rotating speed of the impeller and the
casing geometry must be determined accurately.
Many experimental and numerical studies have been
conducted on the liquid flow through a multistage
centrifugal pump varying one key parameter at a
time (Among other things, La Roche-Carrier et al.,
2013; Miyano et al., 2008; Kawashima et al., 2008,
Gantar et al., 2002). In this study, a concept
consisting of varying three key parameters of
multistage centrifugal pumps at a time was used to
identify parameters to lead to the best design and
performances of multistage centrifugal pumps. Thus,
the following parameters were varied at a time for
the five- and six-stage centrifugal pumps: a) the
numbers of impeller blades (6, 7 and 8), b) the outlet
angle of the impeller blade (19.81°, 21.24° et
25.38°), and c) the impeller width blade (4 mm, 5
mm and 7 mm).
2 GOVERNING EQUATIONS
Fig. 1 shows the domain fluids of five- and six-stage
centrifugal pumps considered in this research work
to run the numerical simulations.
Figure 1: Domain fluids of five- and six-stage pumps.
The following assumptions were made for the
governing equations for liquid flow in the five- and
six-stage centrifugal pumps: (i) a steady state, three-
dimensional and turbulence flow using the k-
model was assumed; (ii) it was an incompressible
liquid; (iii) it was a Newtonian liquid; and (iv) the
liquid’s thermophysical properties were constant
with the temperature.
To account for these assumptions, the theoretical
analysis of the liquid flow in the impeller passages,
diffuser vane passages and diffuser return vane
passages was based on the continuity and
Navier-Stokes equations (Ansys inc., 2011). For the
three-dimensional liquid flow through these five-
and six-stage centrifugal pumps as shown in Fig. 1,
178
Kerbouci L. and Dituba Ngoma G..
Parametric Study of Liquid Flow in Five- and Six-stage Centrifugal Pumps.
DOI: 10.5220/0005543601780182
In Proceedings of the 5th International Conference on Simulation and Modeling Methodologies, Technologies and Applications (SIMULTECH-2015),
pages 178-182
ISBN: 978-989-758-120-5
Copyright
c
2015 SCITEPRESS (Science and Technology Publications, Lda.)