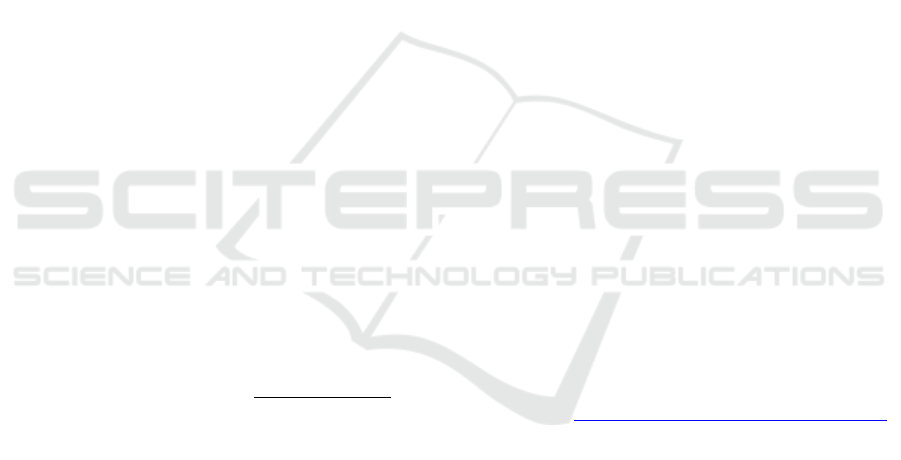
measurements and products (such as electron density
profiles) can be available from the Taiwan Analysis
Center for COSMIC (TACC, http://
tacc.cwb.gov.tw/en/) and the COSMIC Data
Analysis and Archive Center (CDAAC,
http://www.cosmic.ucar.edu/cdaac/).
6 CONCLUSIONS
Only a few examples illustrate the possibility of
obtaining new results using the resources of the
Internet. For ionospheric studies, the most important
are databases of the vertical sounding, measurements
of TEC by high-orbit navigation satellites,
measurements of plasma frequency by low-orbit
satellites, data of solar and geomagnetic conditions.
The obtained results show the large possibilities of
use of resources of the Internet for study of the
ionosphere and the representation of conditions of
propagation of radio-waves. A series of new
knowledge in this area was obtained.
But there is another side to such use: (1) it is not
always possible to reconcile different data to each
other because of their inaccuracies and ambiguities,
(2) there is a danger in the access closing of certain
countries, as it may be in an emergency situation.
ACKNOWLEDGEMENTS
The author thanks the scientists who provided the
data of SPIDR, satellites DMSP, global maps of
TEC, operation and modification of the IRI model,
Dr A. Karpachev (IZMIRAN, karp@izmiran.ru) for
CHAMP data, Southern Federal University for
finantial support (project N 213.01-11/2014-22).
REFERENCES
Bilitza, D., 2001. International Reference Ionosphere,
Radio Sci., 36(2), 261-275.
Bilitza, D., Reinisch, B.W., 2008. International Reference
Ionosphere 2007: Improvements and New Parameters.
Adv. Space Res., 42, 599-609.
Bilitza, D., Altadill, D., Zhang, Y., Mertens, C., Truhlik,
V., Richards, P., McKinnell, L.-A., Reinisch, B., 2014.
The International Reference Ionosphere 2012 – a
model of international collaboration, J. Space Weather
Space Clim., 4, A07, 12p. DOI:
10.1051/swsc/2014004.
De Franceschi, G., Candidi, M., 2013. GRAPE, GNSS
Research and Application for Polar Environment,
Expert Group of SCAR, Annals of Geophysics, Special
Issue 56. 2. P0215; Edited by Giorgiana,
doi:10.4401/ag-6366.
Fuller-Rowell, T., Araujo-Pradere, E., Minter, C.,
Codrescu, M., Spencer, P., Robertson, D., Jacobson,
A.R., 2006. A new data assimilation product from the
Space Environment Center characterizing the
ionospheric total electron content using real-time GPS
data US-TEC, Radio Sci. 41, RS6003, doi:
10.1029/2005RS003393.
Gulyaeva, T.L., 2011. Storm time behavior of topside
scale height inferred from the ionosphere-
plasmosphere model driven by the F2 layer peak and
GPS-TEC observations. Adv. Space Res., 47, 913-920.
Hoque, M.M., Jakowski, N., 2011. A new global empirical
NmF2 model for operational use in radio systems.
Radio Sci., 46, RS6015, 1-13.
Houminer, Z., Soicher H., 1996. Improved short –term
predictions of foF2 using GPS time delay
measurements. Radio Sci., 31(5), 1099-1108.
Jakowski, N., Hoque, M.M., Mayer, C., 2011. A new
global TEC model for estimating transionospheric
radio wave propagation errors. Journal of Geodesy,
85(12), 965-974.
Khattatov, B., Murphy, M., Gnedin, M., Sheffel, J.,
Adams, J., Cruickshank, B., Yudin, V., Fuller-Rowell,
T., Retterer, J. 2005. Ionospheric nowcasting via
assimilation of GPS measurements of ionospheric
electron content in a global physics-based time-
dependent model. Q.J.R.Meteorol. Soc., 131, 3543–
3559.
Maltseva, O., 2014. Choice of the definition method for
the total electron content to describe the conditions in
the ionosphere. Proceedings of Third International
Conference on Telecommunications and Remote
Sensing, Luxemburg, Grand Duchy of Luxemburg, 26-
27 June 2014, 51-61.
Maltseva, O.A., Mozhaeva, N.S., Nikitenko, T.V., 2014.
Validation of the Neustrelitz Global Model according
to the low latitude ionosphere. Adv. Space Res.,54,
463–472, http://dx.doi.org/10.1016/j.asr.2013.11.005.
Maltseva, O.A., Mozhaeva, N.S., Nikitenko, T.V., 2015.
Comparative analysis of two new empirical models
IRI-Plas and NGM (the Neustrelitz Global Model).
Adv. Space Res.,55, 2086–2098.
Maltseva, O., Mozhaeva N., Vinnik E., 2013. Validation
of two new empirical ionospheric models IRI-Plas and
NGM describing conditions of radio wave propagation
in space. Proceedings of Second International
Conference on Telecommunications and Remote
Sensing, Noordwijkerhout, The Netherlands, 11-12
July, 109-118.
Maltseva, O., Mozhaeva, N., Zhbankov, G., 2012. A new
model of the International Reference Ionosphere IRI
for Telecommunication and Navigation Systems.
Proceedings of the First International Conference on
Telecommunication and Remote Sensing, 129-138.
Maltseva, O.A., Poltavsk, O.S., Schlyupkin, A.S., 2007.
The IRI model residual difference and the new method
Fourth International Conference on Telecommunications and Remote Sensing