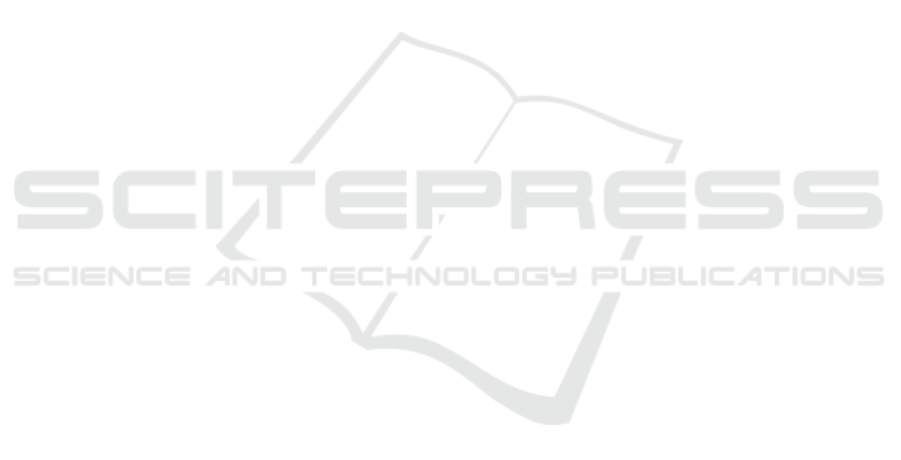
10. Thaut, M.H., Abiru, M.: Rhythmic auditory stimulation in rehabilitation of movement dis-
orders: a review of current research. (2010)
11. McIntosh, G.C., Brown, S.H., Rice, R.R., Thaut, M.H.: Rhythmic auditory-motor facilitation
of gait patterns in patients with parkinson’s disease. Journal of Neurology, Neurosurgery &
Psychiatry 62 (1997) 22–26
12. Suteerawattananon, M., Morris, G., Etnyre, B., Jankovic, J., Protas, E.: Effects of visual and
auditory cues on gait in individuals with parkinson’s disease. Journal of the neurological
sciences 219 (2004) 63–69
13. Roerdink, M., Lamoth, C.J., Kwakkel, G., Van Wieringen, P.C., Beek, P.J.: Gait coordination
after stroke: benefits of acoustically paced treadmill walking. Physical Therapy 87 (2007)
1009–1022
14. Nieuwboer, A., Kwakkel, G., Rochester, L., Jones, D., van Wegen, E., Willems, A.M.,
Chavret, F., Hetherington, V., Baker, K., Lim, I.: Cueing training in the home improves
gait-related mobility in parkinsons disease: the rescue trial. Journal of Neurology, Neuro-
surgery & Psychiatry 78 (2007) 134–140
15. Thaut, M., Leins, A., Rice, R., Argstatter, H., Kenyon, G., McIntosh, G., Bolay, H., Fetter,
M.: Rhythmic auditor y stimulation improves gait more than ndt/bobath training in near-
ambulatory patients early poststroke: a single-blind, randomized trial. Neurorehabilitation
and neural repair 21 (2007) 455–459
16. Bank, P.J., Roerdink, M., Peper, C.: Comparing the efficacy of metronome beeps and step-
ping stones to adjust gait: steps to follow! Experimental brain research 209 (2011) 159–169
17. Storms, R., Zyda, M.: Interactions in perceived quality of auditory-visual displays. Presence
9 (2000) 557–580
18. Sanders Jr, R.D.: The effect of sound delivery methods on a users sense of presence in a
virtual environment. PhD thesis, Naval Postgraduate School (2002)
19. Chueng, P., Marsden, P.: Designing auditory spaces to support sense of place: the role of ex-
pectation. In: CSCW Workshop: The Role of Place in Shaping Virtual Community. Citeseer,
Citeseer (2002)
20. Freeman, J., Lessiter, J.: Hear there & everywhere: the effects of multi-channel audio on
presence. In: Proceedings of ICAD. (2001) 231–234
21. V
¨
astfj
¨
all, D.: The subjective sense of presence, emotion recognition, and experienced emo-
tions in auditory virtual environments. CyberPsychology & Behavior 6 (2003) 181–188
22. Larsson, P., V
¨
astfj
¨
all, D., Kleiner, M.: Perception of self-motion and presence in auditory
virtual environments. In: Proceedings of Seventh Annual Workshop Presence 2004. (2004)
252–258
23. Kapralos, B., Zikovitz, D., Jenkin, M.R., Harris, L.R.: Auditory cues in the perception of self
motion. In: Audio Engineering Society Convention 116, Audio Engineering Society (2004)
24. V
¨
aljam
¨
ae, A., Larsson, P., V
¨
astfj
¨
all, D., Kleiner, M.: Travelling without moving: Auditory
scene cues for translational self-motion. In: Proceedings of ICAD05. (2005)
25. Tonetto, P.L.M., Klanovicz, C.P., Spence, P.C.: Modifying action sounds influences peoples
emotional responses and bodily sensations. i-Perception 5 (2014) 153–163
26. Bresin, R., de Witt, A., Papetti, S., Civolani, M., Fontana, F.: Expressive sonification of foot-
step sounds. In: Proceedings of ISon 2010: 3rd Interactive Sonification Workshop. (2010)
51–54
27. Rocchesso, D., Bresin, R., Fernstrom, M.: Sounding objects. MultiMedia, IEEE 10 (2003)
42–52
28. Avanzini, F., Rocchesso, D.: Modeling collision sounds: Non-linear contact force. In: Proc.
COST-G6 Conf. Digital Audio Effects (DAFx-01). (2001) 61–66
29. Cook, P.: Physically Informed Sonic Modeling (PhISM): Synthesis of Percussive Sounds.
Computer Music Journal 21 (1997) 38–49
22
EPS Lisbon January 2015 2015 - European Project Space on Intelligent Systems, Pattern Recognition and Biomedical Systems
22