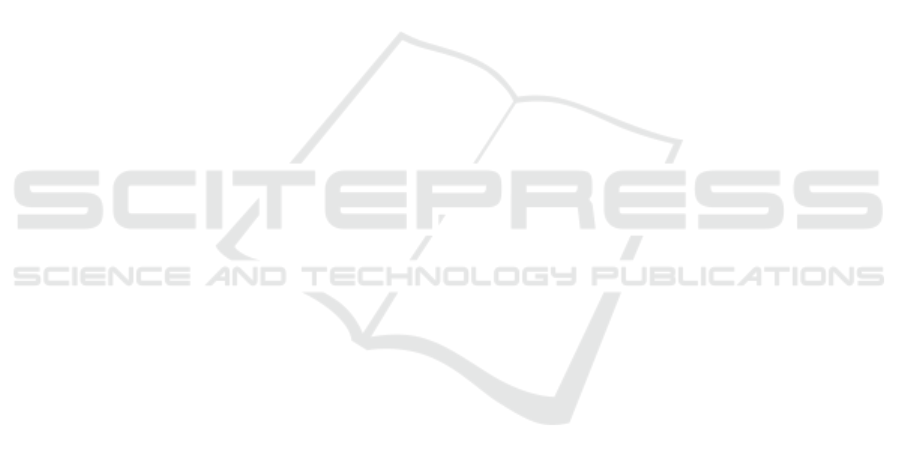
14. Thomsen, C.L., Nielsen, F.D., Johansen, J., Pedersen, C., Moselund, P.M., Møller, U.,
Sørensen, S.T., Larsen, C., Bang, O.: New horizons for supercontinuum light sources:
from UV to mid-IR. In: SPIE OPTO, International Society for Optics and Photonics (2013)
86370T
15. Moller, U., Bang, O.: Intensity noise of normal-pumped picosecond supercontinuum gener-
ation. In: Lasers and Electro-Optics Europe (CLEO EUROPE/IQEC), 2013 Conference on
and International Quantum Electronics Conference, IEEE (2013) 1
16. Kubat, I., Agger, C., Moselund, P., Bang, O.: Mid-infrared supercontinuum generation in ta-
pered ZBLAN fiber with a standard Erbium mode-locked fiber laser. In: Lasers and Electro-
Optics Europe (CLEO EUROPE/IQEC), 2013 Conference on and International Quantum
Electronics Conference, IEEE (2013) 1
17. Kubat, I., Agger, C., Moselund, P.M., Bang, O.: Optimized ZBLAN fiber for efficient and
broadband mid-infrared supercontinuum generation through direct pumping at 1550nm. In:
1st International Workshop on Spatio-Temporal Complexity in Optical Fibers. (2013)
18. Kubat, I., Agger, C.S., Moselund, P.M., Bang, O.: Mid-infrared supercontinuum generation
to 4.5 µm in uniform and tapered ZBLAN step-index fibers by direct pumping at 1064 or
1550 nm. JOSA B 30 (2013) 2743–2757
19. Moselund, P., Petersen, C., Leick, L., Seidelin Dam, J., Tidemand-Lichtenberg, P., Pedersen,
C.: Highly Stable, All-fiber, High Power ZBLAN Supercontinuum Source Reaching 4.75
µm used for Nanosecond mid-IR Spectroscopy. In: Advanced Solid State Lasers, Optical
Society of America (2013) JTh5A–9
20. Møller, U.V., Sørensen, S.T., Petersen, C.R., Kubat, I., Moselund, P.M., Bang, O.: Supercon-
tinuum generation from ultraviolet to mid-infrared. (In: 15th Conference on Optical Fibers
and Their Applications (OFTA 2014))
21. Kubat, I., Rosenberg Petersen, C., Møller, U.V., Seddon, A., Benson, T., Brilland, L., M
´
echin,
D., Moselund, P.M., Bang, O.: Thulium pumped mid-infrared 0.9–9µm supercontinuum
generation in concatenated fluoride and chalcogenide glass fibers. Optics express 22 (2014)
3959–3967
22. Kubat, I., Petersen, C.R., Møller, U., Seddon, A., Benson, T., Brilland, L., M
´
echin, D.,
Moselund, P., Bang, O.: Mid-infrared supercontinuum generation in concatenated fluoride
and chalcogenide glass fibers covering more than three octaves. In: CLEO: Science and
Innovations, Optical Society of America (2014) STh3N–1
23. Kubat, I., Agger, C.S., Møller, U., Seddon, A.B., Tang, Z., Sujecki, S., Benson, T.M., Furniss,
D., Lamrini, S., Scholle, K., et al.: Mid-infrared supercontinuum generation to 12.5 µm in
large na chalcogenide step-index fibres pumped at 4.5 µm. Optics express 22 (2014) 19169–
19182
24. Petersen, C.R., Møller, U., Kubat, I., Zhou, B., Dupont, S., Ramsay, J., Benson, T., Sujecki,
S., Abdel-Moneim, N., Tang, Z., et al.: Mid-infrared supercontinuum covering the 1.4–13.3
µm molecular fingerprint region using ultra-high NA chalcogenide step-index fibre. Nature
Photonics 8 (2014) 830–834
25. Martijn, H., Asplund, C., von W
¨
urtemberg, R.M., Malm, H.: High performance MWIR
type-II superlattice detectors. In: Proc. of SPIE Vol. Volume 8704. (2013) 87040Z–1
26. Kastl, L., Rommel, C.E., Kemper, B., Schnekenburger, J.: Standardized cell samples for
midir technology development. In: SPIE BiOS, International Society for Optics and Photon-
ics (2015) 931507
27. Naranjo, V., Villanueva, E., Lloyd, G.R., Stone, N., Lopez-Mir, F., Alcaniz, M.: Stained
and infrared image registration as first step for cancer detection. In: Biomedical and Health
Informatics (BHI), 2014 IEEE-EMBS International Conference on, IEEE (2014) 420–423
28. L
´
opez-Mir, F., Naranjo, V., Morales, S., Angulo, J.: Probability density function of object
contours using regional regularized stochastic watershed. In: Image Processing (ICIP), 2014
IEEE International Conference on, IEEE (2014) 4762–4766
68
EPS Lisbon January 2015 2015 - European Project Space on Intelligent Systems, Pattern Recognition and Biomedical Systems
68