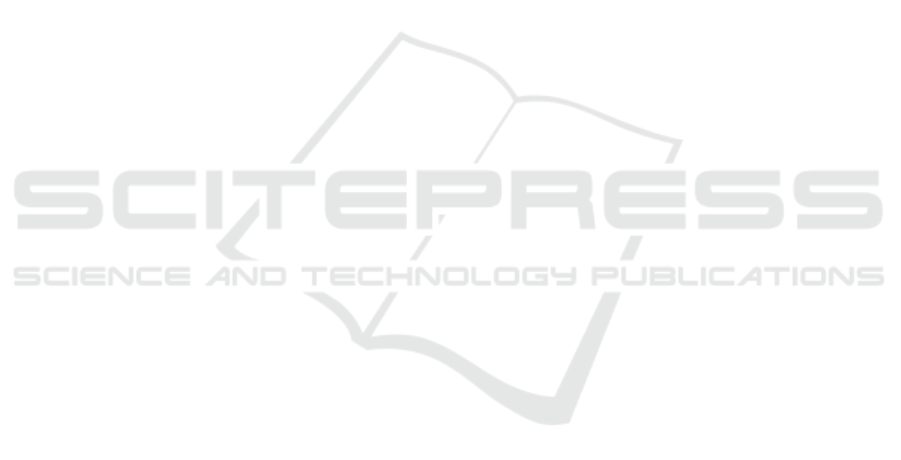
A cognitive mobile robot should therefore explic-
itly include such interworking capabilities, which are
not found in present models for artificial entities. The
model presented in this article fills this gap. Illus-
trative examples show the role the cognitive phases
of this model have in the above two classes of multi-
robot social interaction. Specifically, this article dis-
cussed with the aid of examples how self-awareness
can be exploited for self-organisation by detecting
and managing behavioural anomalies, i.e., abnormal-
ities in group dynamics.
The cognitive phases of the present model are in-
spired by both artificial and natural cognitive enti-
ties. This makes such a holistic model suitable for use
not only for machine-machine, but also for human-
machine interaction.
Interoperability across diverse operational do-
mains and system design reuse across different appli-
cation domains are both timely topics, and for that
robust specifications of functionalities and interfaces
to suit the above goals are needed. This article aims
at this target.
It is evident that explicitly considering social in-
teraction in the model for an intelligent entity brings
advantages to flexible design and specifications. A
comparative evaluation of the proposed model has
been given here. The evaluation of the concepts dis-
cussed in this article by implementation into mobile
robots is part of our future work in this area.
ACKNOWLEDGEMENTS
The authors would like to thank Infotech Oulu for the
financial support.
REFERENCES
Albus, J. (1991). Outline for a theory of intelligence. IEEE
Trans. Syst. Man Cybernet., 21(3):473–509.
Anderson, J., Bothell, D., Byrne, M., Douglass, S., Lebiere,
C., and Qin, Y. (2004). An integrated theory of the
mind. Psychol. Rev., 111(4):1036–1060.
Artz, A. and Armour-Thomas, E. (1992). Development of a
cognitive-metacognitive framework for protocol anal-
ysis of mathematical problem solving in small groups.
Cognition and Instruction, 9(2):137–175.
Brooks, R. (1986). A robust layered control system for a
mobile robot. IEEE J. Robot. Autom., RA-2(1):14–23.
Bryant, D. (2004). Modernizing our cognitive model. In
Command Control Res. Technol. Symp., pages 1–14,
San Diego, CA, US.
Celentano, U. (2014). Dependable cognitive wireless net-
working: Modelling and design. Number 488. Acta
Universitatis Ouluensis Series C: Technica.
Celentano, U. and R
¨
oning, J. (ms). Modelling interactive
cognitive entities. Manuscript.
Dobson, S., Denazis, S., Fern
´
andez, A., Gaiti, D., Gelenbe,
E., Massacci, F., Nixon, P., Saffre, F., Schmidt, N., and
Zambonelli, F. (2006). A survey of autonomic com-
munications. ACM Trans. Autonomous Adapt. Syst.,
1(2):223–259.
Dupourqu
´
e, V. (1984). A robot operating system. In IEEE
Int. Conf. Robot. Autom., volume 1, Atlanta, GA, US.
Kephart, J. and Chess, D. (2003). The vision of autonomic
computing. IEEE Comput., 36(1):41–50.
Kerr, J. and Nickels, K. (2012). Robot operating systems:
Bridging the gap between human and robot. In South-
eastern Symp. Syst. Theory (SSST), pages 99–104,
Jacksonville, FL, US.
Kieras, D. and Meyer, D. (1997). An overview of the EPIC
architecture for cognition and performance with appli-
cation to human-computer interaction. Hum.-Comput.
Interact., 12:391–438.
La, H., Lim, R., and Sheng, W. (2015). Multirobot coop-
erative learning for predator avoidance. IEEE Trans.
Control Syst. Technol., 23(1):52–63.
Langley, P., Laird, J., and Rogers, S. (2009). Cognitive ar-
chitectures: Research issues and challenges. Cogn.
Sys. Res., (10):141–160.
Langley, P., Newell, A., and Rosenbloom, P. (1987). Soar:
An architecture for general intelligence. Artif. Intell.,
33(1):1–64.
M
¨
aenp
¨
a
¨
a, T., Tikanm
¨
aki, A., Riekki, J., and R
¨
oning, J.
(2004). A distributed architecture for executing com-
plex tasks with multiple robots. In IEEE Int. Conf.
Robot. Autom. (ICRA), pages 3449–3455, New Or-
leans, LA, US.
Mitola, J. (2000). Cognitive radio: An integrated agent
architecture for software defined radio. Kungliga
Tekniska H
¨
ogskola (KTH), Stockholm.
Nilsson, N., editor (1984). Shakey the robot. Number Tech-
nical Note 323. Artificial Intelligence Center, SRI In-
ternational, Menlo Park, CA.
Peschl, M., R
¨
oning, J., and Link, N. (2012). Human integra-
tion in task-driven flexible manufacturing systems. In
Katalinic, B., editor, Ann. DAAAM 2012 & Proc. 23rd
Int. DAAAM Symp., pages 85–88, Zadar, Croatia.
Polya, G. (1957). How to solve it: A new aspect of math-
ematical method. Doubleday Anchor Books, Garden
City, NY, 2nd edition.
Quigley, M., Gerkey, B., Conley, K., Faust, J., Foote, T.,
Leibs, J., Berger, E., Wheeler, R., and Ng, A. (2009).
ROS: an open-source robot operating system. In ICRA
Workshop Open Source Software, Kobe, Japan.
Rein, K., Schade, U., and Hieb, M. (2009). Battle man-
agement language (BML) as an enabler. In IEEE Int.
Conf. Commun. (ICC), Dresden, Germany.
Remmersmann, T., Br
¨
uggemann, B., and Miłosław, F.
(2010). Robots to the ground. In Mil. Commun. In-
form. Systems Conf. (MCC), pages 61–68, Warsaw,
Poland.
ICAART 2016 - 8th International Conference on Agents and Artificial Intelligence
124