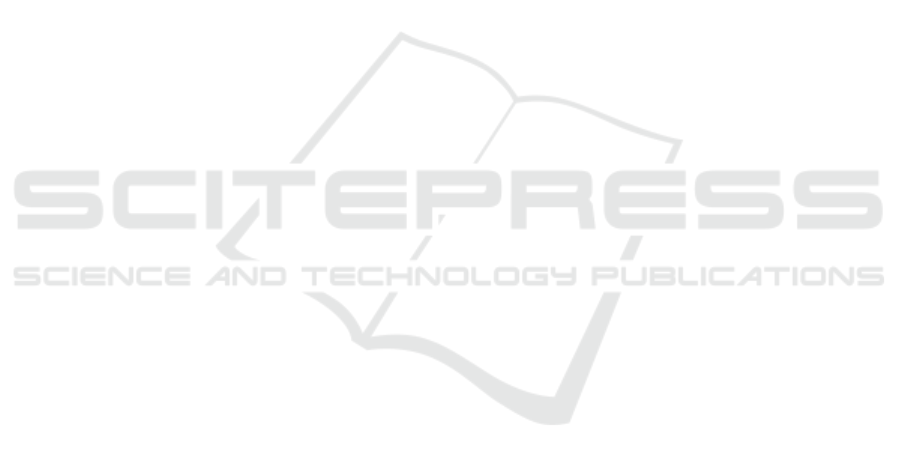
tions obtained from EVM and DEC are in agree-
ment. For the remaining VBGs, in which higher tol-
erated crosstalk levels are estimated, a maximum dif-
ference of 1.2 dB between both estimations is noticed.
Hence, we can conclude that the increase of the toler-
ated crosstalk level leads to discrepancies between the
EVM and the DEC estimations, as the BER estimated
from EVM, using Equation 3, looses accuracy.
4 CONCLUSIONS
In this work, the tolerance to in-band crosstalk of
the VC-assisted DD MB-OFDM system has been as-
sessed numerically. The influence of the VBPR and
VBG of the interferer on the in-band crosstalk toler-
ance has been analyzed.
Our results show that the impact of in-band
crosstalk on the DD OFDM system performance di-
minishes with the increase of the VBPR of the inter-
ferer. The increase of the power of the interferer VC
leads to a power reduction of the interferer OFDM
band, hence, causing less interference on the selected
signal.
The influence of the VBG of the interfering sig-
nal on the in-band tolerance has been evaluated con-
sidering two different scenarios. In scenario (a), the
frequency of the VCs of the selected signal and inter-
ferer are equal. In this case, the tolerance to in-band
crosstalk reduces with the amount of superposition of
interferer and selected OFDM bands. Larger VBG
leads to a reduction of the number of subcarriers that
are affected by crosstalk and the system performance
improves. When the VBG is equal to the OFDM sig-
nal bandwidth, the in-band crosstalk has no influence
on the DD OFDM receiver performance degradation.
In scenario (b), the central frequencies of the se-
lected signal and interferer bands are equal. In this
case, the tolerance to in-band crosstalk is severely di-
minished for a VBG of 83.6 MHz, leading to a tol-
erated crosstalk level that is about 11 dB lower that
the ones obtained for very narrow VBGs. This effect
is due to the detection of the interferer VC on the se-
lected signal and leads to a strong performance degra-
dation. As the VBG of the interferer becomes larger,
the BS filtering reduces the power of the VC of the
interferer and the performance of the DD OFDM re-
ceiver is improved.
The comparison between the numerical results ob-
tained from the DEC and EVM methods shows that,
for higher crosstalk levels, a maximum difference of
2 dB between both methods estimations has been no-
ticed. When the tolerated crosstalk level is lower
than −30 dB, the EVM and the DEC estimations are
in agreement. Hence, we can conclude that higher
crosstalk levels lead to an inaccurate BER estima-
tions.
ACKNOWLEDGEMENTS
This work was supported by Fundac¸
˜
ao para a Ci
ˆ
encia
e Tecnologia (FCT) from Portugal through Project
MORFEUS-PTDC/EEITEL/2573/2012. The project
UID/EEA/50008/2013 is also acknowledged.
REFERENCES
Alves, T., Alberto, A., and Cartaxo, A. (2014). Direct-
Detection Multi-Band OFDM Metro Networks Em-
ploying Virtual Carriers and Low Receiver Band-
width. Opt. Fiber Commun. Conf.
Alves, T. and Cartaxo, A. (2015). High Granularity Multi-
band OFDM Virtual Carrier-assisted Direct-Detection
Metro Networks. 33(1):42–54, paper Tu3G.5.
Alves, T. and Cartaxo, A. (2010). Analysis of Methods of
Performance Evaluation of Direct-Detection OFDM
Communication Systems. Fiber and Integrated Op-
tics, 29(3):170 – 186.
Nezamalhosseini, S., Chen, L., Zhuge, Q., Malekiha, M.,
Marvasti, F., and Plant, D. (2013). Theoretical and Ex-
perimental Investigation of Direct Detection Optical
OFDM Transmission using Beat Interference Cancel-
lation Receiver. Opt. Express, 21(13):15237–15246.
Peng, W., Zhang, B., Feng, K., Wu, X., Willner, A., and Chi,
S. (2009). Spectrally Efficient Direct-Detected OFDM
Transmission Incorporating a Tunable Frequency Gap
and an Iterative Detection Techniques. J. Light. Tech-
nol., 27(24):5723–5735.
Rebola, J. and Cartaxo, A. (2014). Impact of In-Band
Crosstalk Due to Mixed Modulation Formats with
Multiple Line Rates on Direct-Detection OFDM Opti-
cal Networks Performance. In Int. Conf. Transparent
Opt. Networks, pages 14–17.
Shafik, R., Rahman, S., and Islam, R. (2006). On the Ex-
tended Relationships Among EVM, BER and SNR as
Performance Metrics. In International Conference on
Electrical and Computer Engineering (ICECE), pages
408–411.
Winzer, P., Gnauck, A., Konczykowska, A., Jorge, F., and
Dupuy, J. (2011). Penalties from In-Band Crosstalk
for Advanced Optical Modulation Formats. In Euro-
pean Conference and Exhibition on Optical Commu-
nication (ECOC), paper Tu.5.B.7.
Tolerance to in-Band Crosstalk of Virtual Carrier-assisted Direct Detection Multi-Band OFDM Systems
39