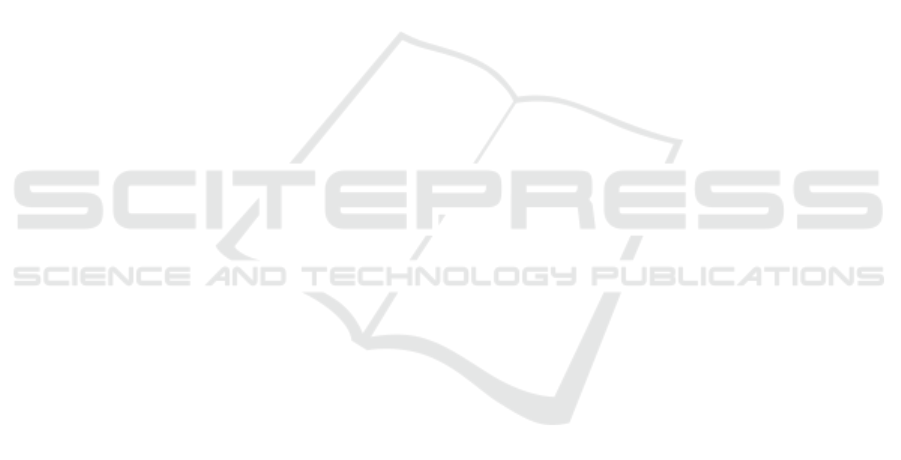
High Perfomance Integrated Temperature Sensor based on
Amorphous Silicon Diode for Photonics on CMOS
Sandro Rao, Giovanni Pangallo and Francesco Della Corte
Università degli Studi “Mediterranea” - DIIES - Via Graziella Feo di Vito 89122, Reggio Calabria, Italy
Keywords: p-i-n diode, Temperature Sensor, Amorphous Silicon, Photonic Integrated Circuit.
Abstract: A temperature sensor based on a photonic layer-integrated hydrogenated amorphous silicon p-i-n diode is
presented. The linear dependence of the voltage drop across the forward-biased diode on temperature, in a
range from 30 °C up to 170 °C, has been used for thermal sensing. A high sensitivity of 11.9 mV/°C in a
biasing current range ≈34-40 nA has been measured.
1 INTRODUCTION
Hydrogenated amorphous silicon (a-Si:H) is a
promising platform enabling the desired matching
between electronics and on-chip photonics (Della
Corte, 2013). Thin layers of a-Si:H can be in fact
deposited using the CMOS-compatible low-
temperature plasma-enhanced chemical vapor
deposition technique (LT-PECVD), with no impact at
all on the microelectronic layers. Moreover, a-Si:H
could be deposited on different substrates where
crystalline silicon (c-Si) could not, be it a glass, a
metal, an already processed silicon wafer, or even
plastic.
Generally, on-chip temperature measurements are
explored for thermal variation compensation in many
sensing devices such as humidity, pressure, flow,
stress and gas concentration sensors (Mansoor, 2015).
Moreover, many Si-photonic active devices are
temperature-dependent, namely they are sensible to
the environment temperature variations due to the
large thermo-optic coefficient of Si. The thermal
sensitivity of the resonant wavelength for silicon ring
resonators is, i.e., of 100 pm/°C about (Yamada,
2011) or, to mention just another example, in a Mach
Zehnder (MZ) interferometer the TO effect is
responsible of a wavelength shift of 90 pm/°C
(Selvaraja, 2010). Consequently, such devices are not
practical without thermal compensation. Thermal
challenges need to be resolved in order to advance the
Silicon Photonics for future network-on-chip
interconnection systems.
In this work, a temperature sensor based on a
waveguide-integrated a-Si:H p-i-n diode is presented.
The linear dependence of the voltage drop across the
forward-biased diode on temperature variations from
T=30°C up to 170 °C has been accurately measured.
Similar sensors based on a-Si:H diodes were
already reported in literature showing however
sensitivities lower than 3.3 mV/°C in a temperature
range from T=30 up to 80 °C (De Cesare, 2015).
2 AMORPHOUS SILICON P-I-N
DIODE TEMPERATURE
SENSOR
The diode temperature sensor was integrated in
proximity to a Mach Zehnder interferometer (MZI),
Figure 1(a), and in particular close to the MZI arm
where the propagating optical signal phase shift is
achieved by electric-field induced p-i-n diode carrier
depletion. The schematic layout of the realized device
is shown in Figure 1(d) together with its geometrical
dimensions. More details about the MZI fabrication
are provided in (Rao, 2012).
The schematic cross section of the fabricated a-
Si:H waveguide vertical-integrated p-i-n diode is
shown Figure 1 (c). It consists of an intrinsic a-Si:H
layer, 2-μm-thick, between a p-doped a-SiC:H, 2-μm-
thick, and an n-doped a-SiC:H, 300-nm-thick. The p-
i-n cathode top contact is a 200-nm-thick Al layer.
The active area of device is 2.25·10
-4
cm
2
.
Rao, S., Pangallo, G. and Corte, F.
High Perfomance Integrated Temperature Sensor based on Amorphous Silicon Diode for Photonics on CMOS.
DOI: 10.5220/0005744903690372
In Proceedings of the 4th International Conference on Photonics, Optics and Laser Technology (PHOTOPTICS 2016), pages 371-374
ISBN: 978-989-758-174-8
Copyright
c
2016 by SCITEPRESS – Science and Technology Publications, Lda. All rights reserved
371