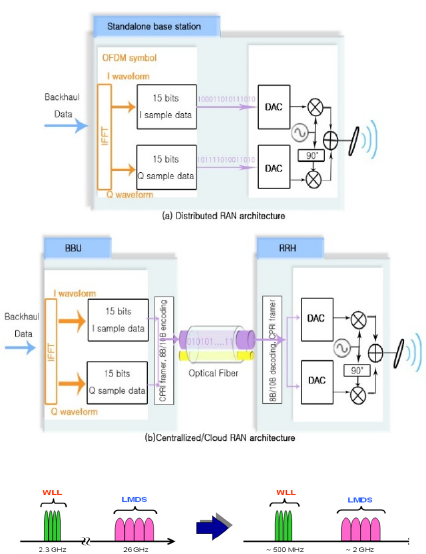
(1)
Actual In fiber
(2)
Figure 1: (1) System architectures of D-RAN (a) vs. C-
RAN (b) and (2) Frequency allocation in backhaul networks
for WLL (wireless local loop) and LMDS (local multipoint
distribution service).
The comparison between distributed RAN (D-RAN)
and C-RAN is shown in Figure 1. While the internal
interface between RU and DU has been defined as the
result of the digitization of the radio signal according
to common public radio interface (CPRI)
(CPRI,v.6.1), an option of optical analog RF wave
transmissions requires much less bandwidth
compared to the CPRI option.
In addition, wireless micro/mm wave systems in
conjunction with optical systems in backhaul
networks have several options for band conversion
between radio and carrier frequencies. Among
system options of baseband, intermediate frequency
(IF), and optical micro/mm-wave transmission, the IF
around 2GHz option is considered as a cost-effective
and efficient solution as shown in frequency
allocations Figure 1 (b).
For wireless systems where signal fading is fatal,
OFDM and SC-FDMA techniques are parallel
transmission schemes modulating data symbols on
multiple carriers simultaneously through an FFT
procedure (Armstrong, 2009). The resulting OFDM
symbol has much longer symbol duration than
temporal dispersion of fading channel. Consequently,
OFDM-based systems are robust to ISI due to multi-
path fading phenomena, and have an additional
advantage of low complexity in implementing an
equalizer.
With all analysis, we adopted a radio over fiber
system in a RF band of a center frequency 2.1GHz
employing OFDM and SC-FDMA techniques.
Subcarrier multiplexing (SCM) was used for
interfacing the optical fiber link with the wireless
system for high speed transmission of signals.
3 SYSTEM CONFIGURATION
Considering downlink application in fronthaul
networks, we elected 16QAM-OFDM and employed
forward error correction (FEC) and pilot tones for
spectral efficiency. Accumulated synchronization
errors were correctable by using some of the
subcarriers in the signal band as pilot tones. The FEC
coding rate was 2/3 and the errors of the sampling
clock in the FEC were less than 10
-4
. The data loaded
subcarriers and the pilot subcarriers were 176 and 24,
respectively.
Signals modulated with 16 QAM scheme and
coded with FEC were transmitted at a data rate of 150
Mbps. Trellis coded modulation (TCM)-OFDM with
an FFT size of 512 was used in multiplexing RF
signals. Spectral efficiency was greater than 2
bits/sec/Hz.
The system configuration is depicted in Figure 2.
The system mainly consists of OFDM/SC-FDMA
modems, baseband signal processing modules, RF
up/down converters, an optical single mode fiber link.
The upper part in Figure 2 corresponds to a DU in a
fronthaul network or a Tx in a backhaul network, and
the lower part does to a RU or a Rx. The system
mainly consists of OFDM/SC-FDMA modems,
baseband signal processing modules, RF up/down
converters, an optical single mode fiber link. The
baseband signals were up converted to RF signals of
2.1GHz by mixing signals from the local oscillator
(LO). The OFDM signals at 2.1 GHz of a center
frequency with the signal bandwidth of 100 MHz
modulate a DFB-LD (distributed feedback laser
diode) with a wavelength of 1550nm and are fed to
20 km of a G.652 fiber. For the system analysis, the
signals were O/E converted in a PD (photo detector)
and then down-converted to the baseband by a LO
and equalized with an automatic gain control (AGC)
mechanism in baseband signal processing.
Digital-to-
analog (D/A) and analog-to-digital (A/D) conversion
were carried out as well. In the baseband processing