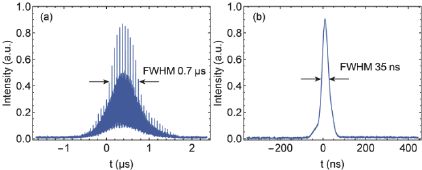
power, the pulse energy was calculated to be 0.46
nJ.
As mentioned above, limited by the peak power
clamping effect, the output pulses were transformed
from the single pulse state to pulse bundle state upon
the pump power was increased to around 240 mW.
With the increasing pump power, the number of the
pulses in single pulse bundle increased accordingly,
while the repetition rate of the pulse bundle
remained at the fundamental frequency 16 MHz. Up
to 5-pulse-bundle was formed with the pump power
of 410 mW. In this case, the output average power
reached 48 mW, and the corresponding energy of the
pulse bundle is calculated to be 3 nJ. The inner pulse
width is measured to be ~200 ps, which is limited by
the bandwidth of the oscilloscope. The pulse
intervals between the inner pulses are not quite
stable, dither around 2.2 ns with a timing jitter of ~
200 ps.
The various mode-locking regimes demonstrated
above were based on the frequency shifting function
of the AOM, where the modulator was not actually
modulated. When the AOM is employed as the intra-
cavity intensity modulator to switch the Q-factor of
the cavity (i.e. the cavity loss), flexible Q-switching
and Q-switched mode-locking operation can also be
facilely achieved. In this case, the FBG-pair-based
laser cavity was adopted and we used an electrical
pulse generator to modulate the power of the RF
driver which is linearly proportional to the insertion
loss of the AOM. The repetition rate and duty cycle
(laser on time/laser off time) of the modulation
signal can be tuned in wide ranges, which will
directly affect the characteristics of the Q-switched
output pulses.
Under the modulation signal of large duty cycle
(typically >50%, dependent on the repetition rate),
the laser can produce Q-switched mode-locked
pulses. Figure 3(a) shows the output pulse
waveforms under the pump power of 1.1 W and the
modulation signal of 100 kHz repetition rate, 60%
duty cycle. The pulse has a Q-switched pulse
envelope with 0.7 μs duration and 100 kHz
repetition rate, and contains a train of ~30 mode-
locked inner-pulses spaced at the laser cavity round-
trip. There are also many noisy minor pulses filled in
the spacing between the mode-locked inner-pulses.
This phenomenon was also observed in Yb-doped
FSF fiber laser (Heidt et al., 2007), as well as
common Q-switched fiber lasers and gain-switched
fiber lasers (Swiderski and Michalska, 2013, Eckerle
et al., 2012). We believe this Q-switched mode
locking regime is initiated by the combined effect of
FSF and Q-switching (Heidt et al., 2007).
Figure 3: The oscilloscope traces of the output pulse
waveforms (a) in Q-switched mode locking regime and (b)
short pulse Q-switched regime.
The mode-locking sub-pulses can be eliminated by
decreasing the duty cycle of the modulation signal
until the laser-on time is less than the building time
of the mode-locked pulses. Then the pulse
waveforms become smooth and stable, indicating a
regular Q-switching regime. In this regime, the
repetition rate follows the modulation signal, and the
pulse width is related to the repetition rate, the duty
cycle and the pump power. With the repetition rate
fixed at 1 kHz and the pump power at 1.1 W, the
shortest pulse width 35 ns can be obtained with the
duty cycle set at 1%, as depicted in Fig. 6(b). In this
case, the output power reached 36 mW. The pulse
energy was 36 μJ, and the peak power was nearly 1
kW. The highest pulse energy 78 μJ can be obtained
under the duty cycle of 50%, and the corresponding
pulse width was 47 ns, and the peak power was 1.65
kW. Under the highest pump power of 1.1 W, with
the flexible adjustment of the modulation repetition
rate and duty cycle, the repetition rate of the Q-
switched pulses can be adjusted in a wide range
(from 1 kHz to >500 kHz) and the pulse duration
can be tuned from 35 ns to 770 ns.
REFERENCES
Eckerle, M., Kieleck, C., Świderski, J., Jackson, S. D.,
Maz, G. & Eichhorn, M. 2012. Actively Q-switched
and mode-locked Tm 3+-doped silicate 2 μm fiber
laser for supercontinuum generation in fluoride fiber.
Optics letters, 37, 512-514.
Heidt, A., Burger, J., Maran, J.-N. & Traynor, N. 2007.
High power and high energy ultrashort pulse
generation with a frequency shifted feedback fiber
laser. Optics express, 15, 15892-15897.
Swiderski, J. & Michalska, M. 2013. Generation of self-
mode-locked resembling pulses in a fast gain-switched
thulium-doped fiber laser. Optics letters, 38, 1624-
1626.